Small Enough
by Alan S. Brown
Make something small enough and it can solve many persistent problems
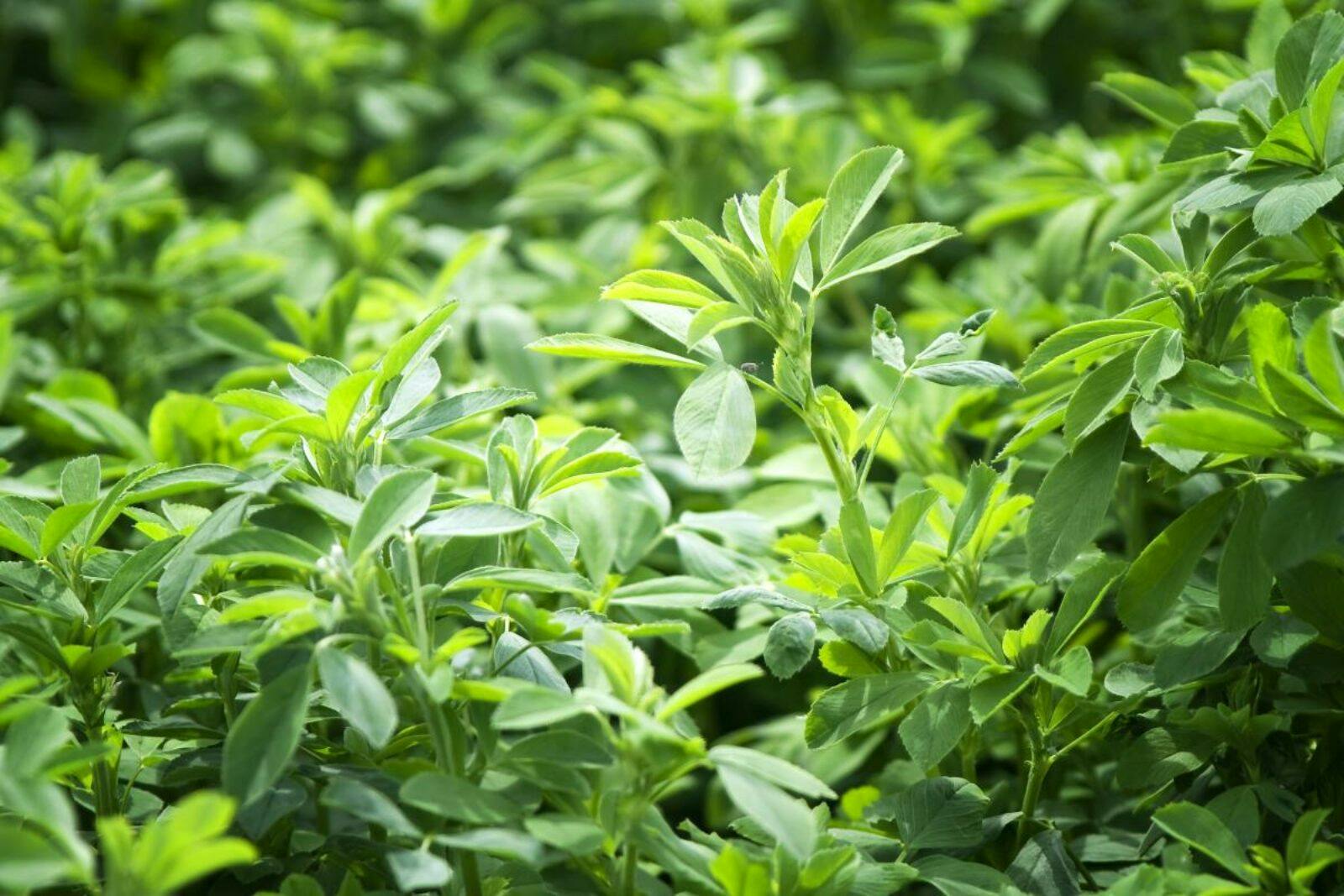
The Author
The Researchers
Make something small enough and it can solve many persistent problems. Take, for example, understanding how our brains work. Researchers have tried to do this in the past by inserting a small metal tube and some electronics into the brain. It was messy, but a dozen or two neurons would eventually grow into it. Researchers could then tell when the neurons sparked and try to guess why. Now, Kavli researchers have developed a tiny light emitter they can implant deep in the brain, that can collect optical information through thousands and perhaps millions of individual neurons at a time, giving us a window onto the brain’s inner workings.
Inside the Brain
The brain is one of nature’s greatest mysteries. Where do our thoughts come from? How do we make memories? What makes us like one thing and not another? The answers are determined by the way our cerebral neurons communicate with one another. In the past, researchers have inserted probes into the brain that measure the electrical sparks of dozens of neurons as they communicate with one another. After they filter out the background noise—an incredibly difficult feat—they use the data to trick out some answers about what our brain is doing. Now, physicist Michael Roukes at the Kavli Nanoscience Institute at Caltech has developed a technique that could let us track from thousands to millions of neurons at a time. “Integrated neurophotonics” combines microchips that emit and record light and genetically modified neurons that express fluorescent markets when lit by the light. Because the system uses optical information, it does not have issues with the background electrical noise of billions of brain cells. The new system may one day provide answers to questions that were unanswerable until now.
DNA identifies urinary tract infections
Urinary tract infections affect 150 million people annually. Most of the time, they are an uncomfortable inconvenience, but they can cause serious complications if they spread to the kidneys. Today, doctors diagnose UTIs with urine cultures, but this is slow and not all pathogens grow in culture. Now a team of three researchers, including biomedical engineering Iwijn De Vlaminck, a member of the Kavli Institute at Cornell, have developed a better way to identify the bacteria that cause these infections so doctors can treat them. It involves sequencing DNA that has escaped from bacterial cells and floats freely in the bloodstream. Ideally, this will enable the researchers to tell exactly which pathogens are present in what organs so they can improve their treatments. The team will test the approach for six months on 300 kidney transplant patients, who are prone to infection and could lose their kidney if not treated early.
Entangled photosynthesis
Remember the basics of photosynthesis? Plants absorb light and use its energy to turn water and carbon dioxide into sugars. But how, exactly, do they do that? What happens when they absorb a photon, a quantum particle of light? Where does it go, and how do they get energy out of it? If we want to use sunlight to manufacture fuels, drugs, and chemicals, we need to know these answers. Two members of the Kavli Energy NanoScience Institute at UC Berkeley, chemists Graham Fleming and Katharine Birgitta Whaley, are trying to tease them out using quantum methods. They use a technique called quantum light spectroscopy, which entangles two photons, so both photons share the same properties even when separated. This makes it possible to measure what happens to a proton when a plant extracts energy from it without harming the plant with measurement instruments.
Motion sets sensor in motion
Over the past few years, engineers have pioneered inexpensive wearable sensors to monitor things like heart rate, body temperature, blood sugar, and metabolic byproducts. This has given medical researchers unparalleled insights into how our bodies change over days or weeks—at least until the batteries wear out. Now medical engineer Wei Gao, a member of the Kavli Nanoscience Institute at Caltech, has developed a device that powers those sensors through movement. It works much the way we generate static electricity by rubbing a balloon against our clothing. In this case, we attach a sandwich of Teflon, copper, and nylon plastic to the torso and a sheet of copper and nylon under the arm. When we move, they rub, producing just enough electricity to power the sensor and send data to a nearby smartphone via Bluetooth.
Valleytronics switches on
Valleytronics may sound like an electronics superstore in L.A., but it is actually a quantum mechanical technique that could lead to super-fast, next-generation electronics. Like most quantum technologies, this one involves subatomic particles, in this case, electrons. Its name comes from the two “valleys” in a material’s energy bands, which are determined by an electron’s orbital angular momentum. If we could find a way to shift all the electrons from one valley to another, we would have the equivalent of a transistor with an on (1) and off (0) state. That has been challenge, but physicist Kin Fai Mak, a member of the Kavli Institute at Cornell for Nanoscale Science, has developed a technique to do it by manipulating the magnetic field on a second material. If valleytronics can be scaled, they would provide smaller faster electronic devices.