Sound Gives Electrons a New Spin
by Alan S. Brown
Gregory David Fuchs, member of the Kavli Institute at Cornell for Nanoscale Science, studies spin physics
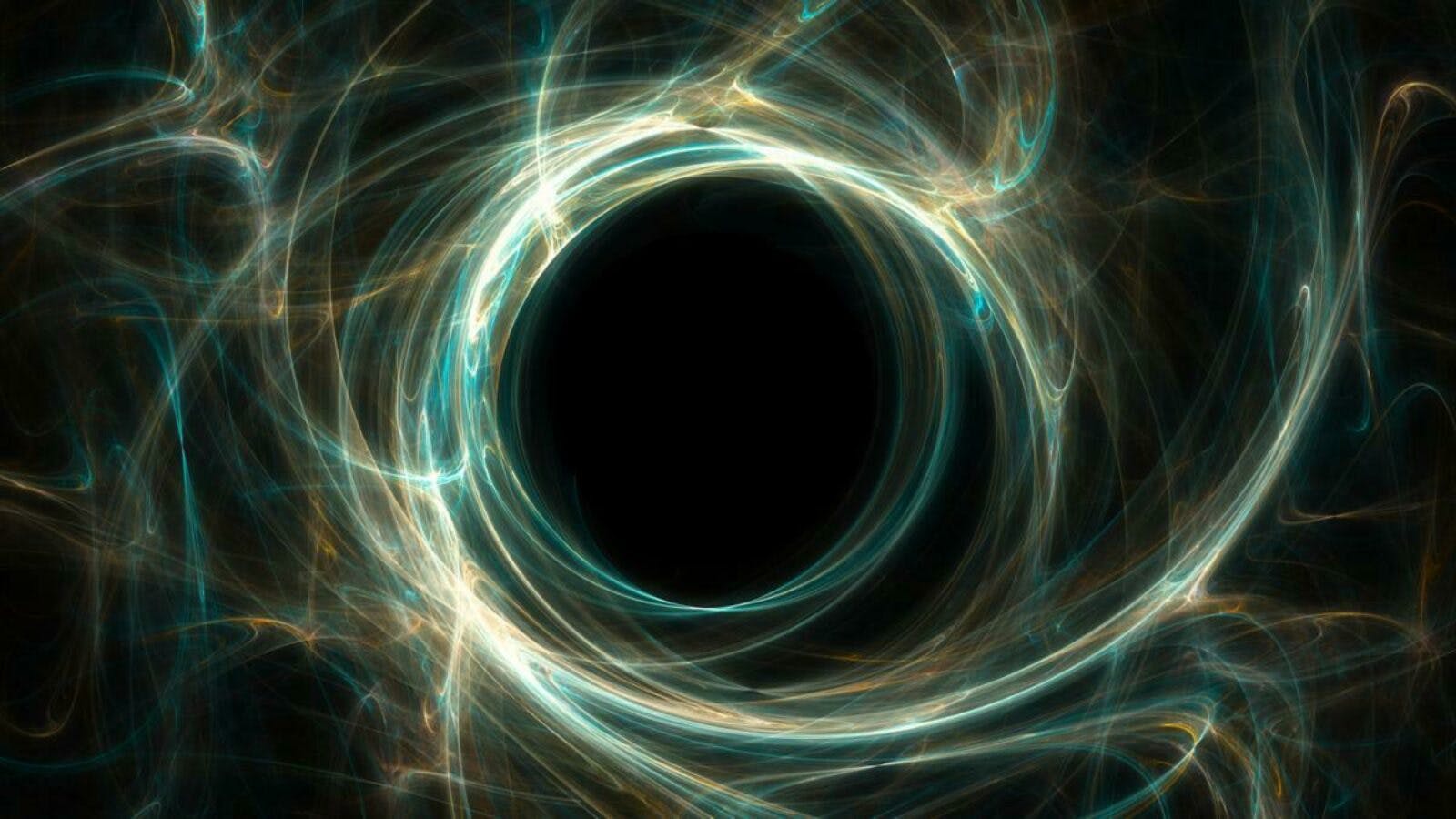
The Author
The Researcher
The spin of an electron is infinitesimally small, the merest whisper of the quantum world. Yet electron spin is the origin of all magnetism, from refrigerator magnets to high-tech hard drives. It influences the position of electrons in atoms, their properties, and the flow of solar winds and beams in particle accelerators.
Because of their quantum nature, electron spins could act like switches to perform calculations in quantum computers, provide security for quantum networks, and map the fluctuations of magnetic fields thousands of times thinner than the width of a sheet of paper.
Yet our understanding of electron spins, like most quantum phenomena, remains elusive. "If want you a classical analog, imagine a ball covered with charges that turns on its axis," said Gregory David Fuchs, an associate professor of applied and engineering physics and member of the Kavli Institute at Cornell for Nanoscale Science, who studies spin physics. "The fact that it's a charged and spinning gives it a magnetic moment.
Of course, spinning electrons are not really spheres. They have no mass and do not really spin, even though they behave like they do. And, since this is the quantum world were everything happens in discrete jumps, the simplest systems do their equivalent of spinning in two directions--up and down.
That is actually a good thing. It turns out that up and down spins are exactly what is needed to build a qubit, the quantum equivalent of a transistor that is the basis of all digital devices. Only a qubit, in addition to up-down spins that are similar to a transistor's on-off states, also has a third position.
This third state is a superposition, where up and down spins overlap. In fact, this superposition can be in both states at once until it is measured. By taking advantage of superpositions, quantum computers can perform calculations that baffle their digital counterparts.
"Electron spin lies at the heart of a lot of the technology that's under development right now," Fuchs said. But to take advantage of it, researchers need a better way to control it. Fuchs thinks he may have one, and it involves putting the squeeze on diamonds.
Vacancies
Fuchs came to spin physics almost by accident. He grew outside Minneapolis-St. Paul, where many neighbors were engineers at 3M. Interested in science but clueless about a career in the field, he majored in education at University of Wisconsin and taught science for five years.
Somewhere along the way, he decided he wanted to do research. After calling several professors, a physicist at University of Minnesota finally gave him a project to do. "He let this high school teacher into his lab," Fuchs said. "And he's a magnetics guy, so that's how I got into magnetics."
Soon, Fuchs was off to graduate school at Cornell, where he earned a Ph.D. while studying nanoscale magnets so small, they often showed quantum behavior. Afterwards, he landed a post-doc with David Awschalom at University of California, Santa Barbara, a leader in spin physics.
At the time, researchers were trying to isolate individual ions to study their spinning electrons. One way to do that was to create a perfect crystal that was missing an atom. This formed a void where they could trap an electron. Fuchs started working on diamond.
"Diamond is a lattice made up of repeating carbon atoms," he explained. "I wanted to find a vacancy where there are no carbon atoms, then replace a neighboring carbon with a nitrogen ion. The carbon acts like an electrical insulator to trap the electron.
"And it has another important property. If you shine a light on it, a single electron will emit a bright red light. You can actually determine the spin of a single electron by the measuring the amount of light it emits."
Best of all, it was cheap (at least as far as scientific instruments are concerned). Most electron spin experiments required big-ticket equipment for high vacuums and near-absolute-zero temperatures. Fuchs could run his experiments all day in air at room temperature with just a laser, microscope, light detector, and a handful of filters and mirrors.
By the time he joined the Cornell faculty in 2011, he had transitioned from nanoscale magnets to single spins. He also idea. He already knew he could manipulate his nitrogen vacancies with light, magnets, electric fields, microwaves. But what about mechanical force?
The big squeeze
When he came to Cornell, Fuchs was asking some basic science questions. How, he wondered, did diamond nitrogen vacancy systems differ from the ion traps that combined cryogenic temperatures with high vacuums?
"The answer is that it has a lattice, which is always vibrating," he recalled. "So I started to think about it in terms of stress. And I wondered, if I squeezed the crystal to push its atoms slightly together, would it do something to the nitrogen vacancy?"
The answer was a resounding, "Yes." In most experiments with nitrogen vacancy centers, researchers shake the electrons from up to down and back with oscillating electromagnetic fields. Fuchs found he could do the same thing by applying pressure mechanically.
The result resembled the tiny vibrating silicon devices cellphones use to lock onto a frequency to communicate with the local base station on a call. They are called high overtone bulk acoustic resonator. In 2013, Fuchs build one using his nitrogen vacancy system.
This opened the door to some interesting applications.
A sense of scale
Now that he has an all-mechanical system to manipulate an electron's quantum states, Fuchs is using it to map magnetic fields.
One of the intrinsic properties of a spinning electron is that it creates a magnetic field. It is very sensitive to other magnetic fields, in much the same way one magnet will repel or attract another. Fuchs is using it to map the magnetic properties of materials with nanoscale precision.
To do this, Fuchs attaches a nitrogen vacancy diamond to the tip of an atomic force microscope, a device that ordinarily runs along the surface of an object like a phonograph needle and measures its topography. By running the microscope's tip a few nanometers above the surface, the nitrogen vacancy can sense magnetic forces of the material below.
"We're doing it at room temperature in air," Fuchs said. "We can study a material's magnetic field with nanometer precision. It works so well, it's amazing."
Fuchs believes the same sensitivity could track slight fluctuations in the Earth's magnetic fields. Such a chip could track the location of a cellphone if its GPS loses contact in a tunnel or among tall buildings. That is a steep challenge.
To go further, Fuchs must find a way to team electron spins so they--and their quantum states--work together. "There is no universal answer to that question," Fuchs said.
Part of the challenge involves scale. To harness spin, it must interact with something big enough to communicate at human scale.
"That is not necessarily an easy thing to figure out," he said. "It's hard to argue that there is anything is more fundamental than an electron spin. You can have these quantum states and a superconductor to carry information, but how do you make them talk to each other?"
Mechanical devices might provide an answer. They give him the flexibility to engineer them to behave in certain ways and perform certain tasks. "It is like a lot of other things in the quantum world, it is doable but hard," he said.
But if he and other spin physicists can tap these fundamental forces, they can move beyond experiments and help shape a new world of computation, computing, sensing, and who knows what else.