Spotlight Roundtable: Witnessing Starbursts in Young Galaxies
by Bruce Lieberman
Three leading scientists discuss how the world’s most powerful radio telescope revealed that the most vigorous bursts of star birth in the cosmos took place much earlier than previously thought.
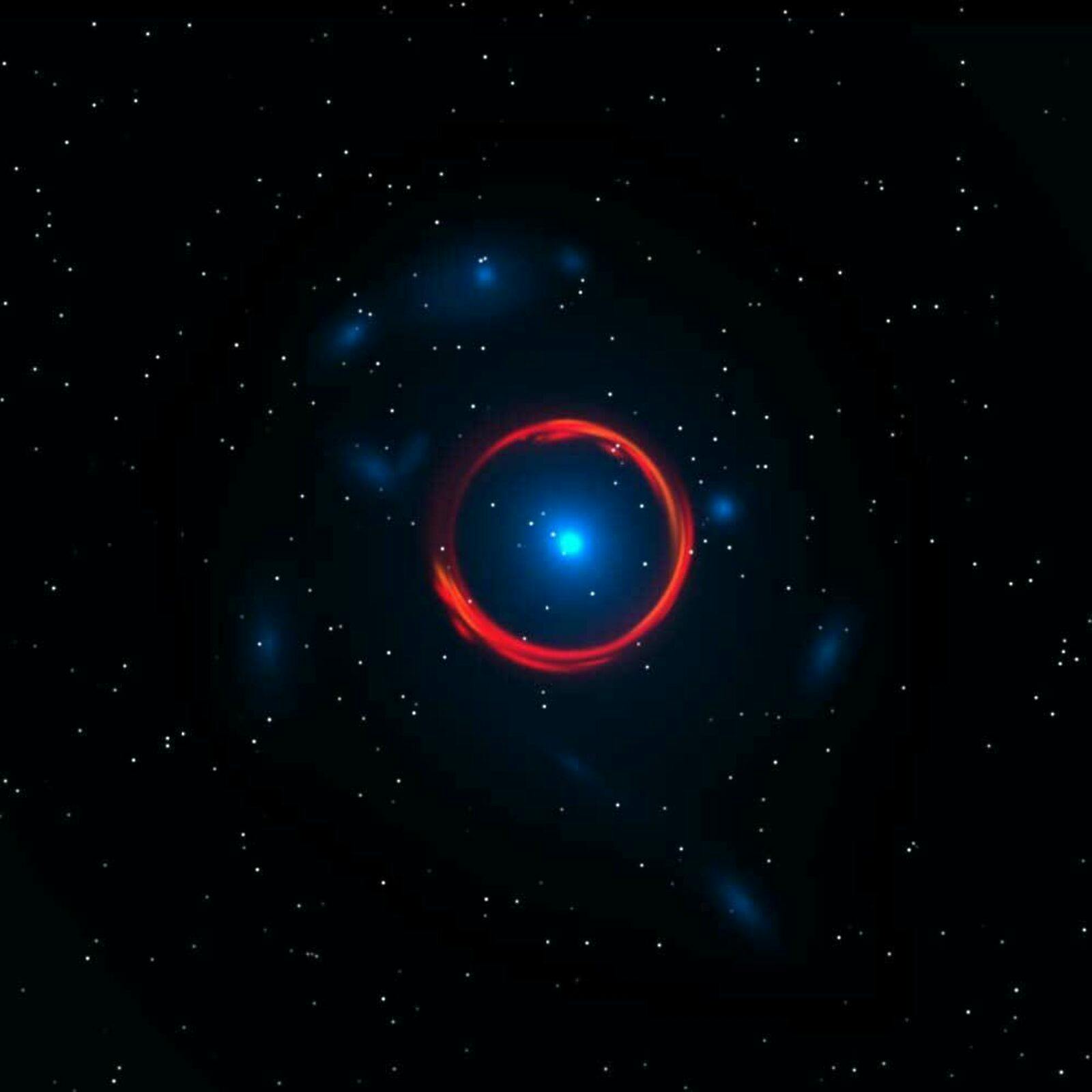
The Author
The Researchers
Now, a multi-national team of astronomers has found that these distant, dusty galaxies were churning out stars much earlier than once believed – as early as one billion years after the Big Bang, nearly 13 billion years ago. Their study was published online on March 13 by the journal Nature. (Press releases: California Institute of Technology, ESO, KICP/University of Chicago, University of Arizona)
Measuring just how far away these galaxies are, and examining the rapid star formation going on inside them, was no trivial feat. Armed with a catalog of galaxies discovered by the South Pole Telescope (SPT), the astronomers used some fortuitous natural phenomena and the great resolving power of the Atacama Large Millimeter/submillimeter Array, or ALMA – an array of radio antennas situated on a high plateau in the Atacama Desert of Northern Chile – to learn about some of the most distant star-forming galaxies.
Three members of the team spoke recently with The Kavli Foundation in a roundtable discussion about their discovery and what they plan next. The participants:
- John E. Carlstrom – Subrahmanyan Chandrasekhar Distinguished Service Professor in the Departments of Astronomy and Astrophysics as well as Physics at the University of Chicago, and Deputy Director of the UChicago’s Kavli Institute for Cosmological Physics (KICP). Prof. Carlstrom is an observational cosmologist who studies the Cosmic Microwave Background (CMB). He is also leader of the 10-meter South Pole Telescope project, which recently completed a survey of 2,500 square degrees of the sky, and is now conducting a survey of the polarization of the CMB.
- Dan P. Marrone – Assistant Professor in the Department of Astronomy at the University of Arizona. Prof. Marrone is interested in galaxy clusters, galaxy formation in the early universe, and the physics of the supermassive black hole in our galaxy, Sagittarius A*.
- Joaquin D. Vieira – Postdoctoral Scholar at the California Institute of Technology and a member of Caltech’s Observational Cosmology Group. Dr. Vieira is interested in studying galaxy evolution at very high redshifts, the first stars and galaxies, and the evolution of large-scale structures in the universe. He is the leader of the group studying the galaxies discovered by the South Pole Telescope.
The following is an edited transcript with remarks added by the participants.
THE KAVLI FOUNDATION: About a third of the galaxies that you observed in your study existed at extremely early times, when the universe was only about one billion years old. You must all have mental picture of what the early universe could have been like. How did your findings change your views about what was actually going on then?
JOAQUIN VIEIRA: My expectations before this study were more focused on what I thought we’d be able to detect, rather than what I thought the universe was like back then. We knew we’d be excited to find anything at a redshift greater than four.
To understand what that redshift measurement means, it’s important to understand, by the time it reaches us, the wavelength of light from very distant galaxies is stretched by the expansion of the universe. The result is that the light spectrum of these galaxies – that is, the rainbow of colors that make up the overall light emitted by each galaxy – has been shifted toward the redder end of the electromagnetic spectrum, from the part that’s visible to our eyes toward longer wavelengths of light that make up the infrared part.
By measuring how much the light from these galaxies has been shifted toward the infrared, we can calculate how far away they are and how far back in time they existed. That’s where the word “redshift” comes from, and higher numbers in the redshift scale correspond to farther distances from Earth, and farther back in time.
TKF: But ALMA has changed all that.
VIEIRA: It’s changed everything. After making our observations with ALMA, we doubled the number of these dusty starburst galaxies above a redshift of four. When we first started planning the redshift survey with ALMA, we had already tried with four other observatories to measure redshifts for the SPT sources – and it was very difficult and very frustrating. We basically had no success at all. We were thinking that if we got just a handful of redshifts with ALMA, we would be really lucky. But in the end we got redshifts for 90-percent of the sources in our survey catalog – out to higher redshifts then we really thought was possible.
JOHN CARLSTROM: We did expect to see bright galaxies in the South Pole Telescope survey, but not the dusty star-forming galaxies that we found. Instead, we thought we’d detect the very bright centers of galaxies where jets of radiation are emitted from black holes. These phenomena, often referred to as radio sources, are pretty well known.
Before our observations with ALMA, Joaquin had catalogued galaxies detected by the South Pole Telescope. When he analyzed the spectra of these objects, he discovered that some of the sources appeared to be dominated by emission from dust. They were not in line with what you would expect from the well known population of radio sources. This was the first clue we were onto something interesting. Then Joaquin discovered there were no counterparts to these galaxies catalogued by infrared surveys of the sky. That was baffling. It meant that they had escaped detection in the infrared surveys. No one had predicted that we would see such a luminous population of dusty galaxies so far back.
DAN MARRONE: I agree with Joaquin and John that our ability to get redshifts for these galaxies was a surprise – and that’s a testament to the power of ALMA. Previously, we had tried making observations at the same wavelengths with the southernmost observatory available, the Submillimeter Array in Hawaii, and we pointed the radio dishes basically at the horizon to watch these galaxies just barely come up over the dirt. We were able to see them, but we had no real hope of getting redshifts for them. We still couldn't really figure out how far away they were. We needed something like ALMA to pull that off.
TKF: John, I wanted to come back to something that you said earlier. You said it was a surprise for you to find these dusty star-forming galaxies at such early times. When we talk about “dusty, star-forming galaxies” do we mean galaxies that show elements other than hydrogen and helium? Like iron, carbon, silicon, etc.?
CARLSTROM: By “dusty” I do mean that. When stars form, they quickly enrich the gas surrounding them with heavier elements. And those elements then form dust particles, and the dust particles absorb the starlight and then re-radiate that as a thermal emission at much longer wavelengths. We refer to that thermal radiation at longer wavelengths as “dusty emission” because it's coming from the dust. But it is actually energy that is generated by the stars that form.
TKF: These early dusty galaxies were churning out stars, as your study says, at a rate of 1,000 stars per year – compared to about one star per year for the Milky Way and other galaxies in modern times. Why do we think that star formation was so much more vigorous in the early universe?
MARRONE: In the early universe, in general, a much larger fraction of the mass of galaxies was in gas. That alone pushes up the star formation rate.
The universe also was much smaller back then, so galaxies were much closer together. As a result, we expect that they were much more likely to have interacted with one another. And collisions between two galaxies will trigger bursts of star formation. Today, because of the expansion of the universe, galaxies are further apart on average and these interactions are much less common.
TKF: In your study, you note that these early galaxies you observed are shining with the energy of a trillion suns but have masses that are much less than that. This suggests that stars burned brighter back then. Was this finding a surprise, and what does it tell us about the character of stars at these early times?
VIEIRA: When stars form, they come in a wide range of masses, from much less than the Sun to tens of times more massive than the Sun. The most massive stars are incredibly bright, but they live very short lives before they become supernovae. The energy output we measure from these galaxies shows us that the most massive stars created in the starburst have not yet used up their fuel and exploded, though they will do so relatively soon.
TKF: The team took advantage of two natural phenomena to observe these galaxies in detail: one was that their light was magnified by the gravity of closer objects – a phenomenon known as gravitational lensing. But there was a second phenomenon that’s a bit harder to understand, and it has to do with the fact that dusty galaxies are not dimmer the farther away they are. Why is this the case?

MARRONE: At wavelengths near 1 millimeter, the spectrum of dust is very interesting. It gets brighter very quickly as you look at shorter and shorter wavelengths. For example, the SPT observes these galaxies at 2 millimeters (and 1.4 and 3). If you look at a dusty galaxy at 2 millimeters and 1 millimeter wavelengths, it will be about 10 times brighter at 1 millimeter than at it is at 2 millimeters wavelength. That’s for looking at the same galaxy (at the same redshift). Now imagine you look at two different galaxies at two different redshifts, but at the same wavelength. The light from the higher redshift (more distant) galaxy will have been stretched more, so you measure the light at, say, 2 millimeters wavelength, but the original wavelength will have been not 1 millimeter, but maybe 0.5 millimeters. It was 100 times brighter when it was emitted. Of course, because it’s further away it looks fainter, just like a light bulb would look fainter across the street instead of in your house. But starting out so much brighter almost perfectly cancels that effect.
This is incredibly valuable for us. You have all these dusty galaxies existing throughout the history of the universe, and no matter how far away they are they're not really getting much dimmer when we look at these wavelengths. So, unlike at optical wavelengths, where distant galaxies get dimmer and dimmer and the deep sky ends up looking black, light from these dusty galaxies creates a mostly diffuse and flat infrared sky. And we call that the Cosmic Infrared Background.
TKF: And that's where ALMA comes in. It’s used to measure the spectra of these dusty galaxies and determine how far away they actually are.
MARRONE: That's right. Because these dusty galaxies do not get any dimmer as they get farther away, we actually don't know where they are. But ALMA’s sensitivity in measuring the spectra of each of these objects allows us to measure how much those spectra are shifted toward the redder end of the spectrum – and obtain precise redshifts.
TKF: Tell me about ALMA. How would you describe it?
CARLSTROM: ALMA is like a very high-powered telescope. Imagine you’re looking through a large telescope; you need a finder scope on the side to see where you’re pointing. That’s because the field of view of the big telescope is so tiny. ALMA, like the big telescope, gives you this incredibly detailed image of whatever you're looking at, plus this beautiful spectroscopic capability. But it only looks a very tiny field of view.
TKF: So ALMA allows you to look much closer at galaxies already identified by the South Pole Telescope, which is being used to survey a large fraction of the sky, correct?

CARLSTROM: Yes. ALMA is most powerful when it’s teamed up with other observatories that conduct surveys of huge swaths of the sky to identify interesting targets worthy of more detailed examination. It enables a detailed examination of extremely distant objects because it operates as an interferometer. That means its effective resolving power isn’t equal to that of one antenna, but to an antenna that is the diameter of the entire array. So ALMA’s 12-meter antennas, collectively, get orders of magnitude higher angular resolution than what you would get from the single 10-meter South Pole Telescope. As a result, ALMA gives us very, very detailed images.
TKF: So ALMA’s role here was critical.
CARLSTOM: ALMA is designed to detect the exact same wavelengths of light in which the dusty galaxies were discovered by the South Pole Telescope. As a result, there was no doubt ALMA was seeing the same galaxies. Also, ALMA gives us very high spectral resolution, so we were able to analyze the light from these galaxies with great precision to identify carbon monoxide and other molecules. That told us about what’s in those galaxies, but more importantly for our study, that level of detail allowed us to measure the shifting of that spectra toward the red part of the spectrum – the redshift. And measuring changes in this part of the spectrum is the way we could tell how far away these objects are.
TKF: The ALMA observatory isn’t completed. Right now it has an array of about 16 antennas, but within a year or so it will have 66. How will this help you in the future?
MARRONE: With little more than a dozen antennas at ALMA, we were able to make very detailed images of these galaxies – and that was after just 2 minutes of observations per galaxy. When we were trying this with The Submillimeter Array in Hawaii – which has only eight antennas, each only half the size as the ones at ALMA – we were observing each galaxy for a couple of hours. Even then, we were not getting anything like the detail that ALMA gave us, though this is a little unfair to the SMA, since it had to look so low on the horizon to see our sources. When ALMA is completed, the observations we obtained for this first study are just going to be trivial. You almost feel bad using ALMA to look at them – the exposures are so fast. You want to give ALMA more of a challenge.
CARLSTROM: I would add that a key science goal for the James Webb Space Telescope is to study the very first galaxies that emerged in the universe. This telescope is regarded as the successor to the Hubble Space Telescope, and it’s anticipated for launch in 2018. The studies we’re doing with ALMA now are allowing us to get to a jumpstart on that whole quest.
TKF: In a way, what you have is the ultimate zoom camera, letting you see details about the early universe once unimaginable. Now that you’ve seen what ALMA can do, what are your next steps?
MARRONE: I'd really like to push ALMA to its full potential and examine individual star-forming regions within these galaxies. One of our galaxies, at a redshift of 5.7, is forming stars at a rate per unit area that’s as high as anything we’ve ever observed in the entire universe. ALMA is going to make it possible for us to pick that apart, to resolve the individual star-forming regions that are giving us those incredible starbursts.
VIEIRA: Now that we have the redshifts for these galaxies, we can dig deeper into the spectra of these galaxies to find out what they’re made of; we can do chemistry with them. We have a sample of about 100 galaxies, and so what we want to do is get redshifts for all of them. Then we can map out the redshift distribution of these sources in an unbiased way. We’ll be looking at individual spectral lines in detail, and examining the chemical makeup of these galaxies – even from region to region within them. Future studies also will help us answer other important questions, such as how they formed. Did they form through mergers, or through the slow accretion of gas? How many stellar generations reside in these galaxies?
These questions are all really exciting, and answering them is eventually going to change our view of the universe. Right now we've only taken the first step.