Stanford Experts on LIGO’s Binary Neutron Star Milestone
(Originally published by Stanford University)
October 16, 2017
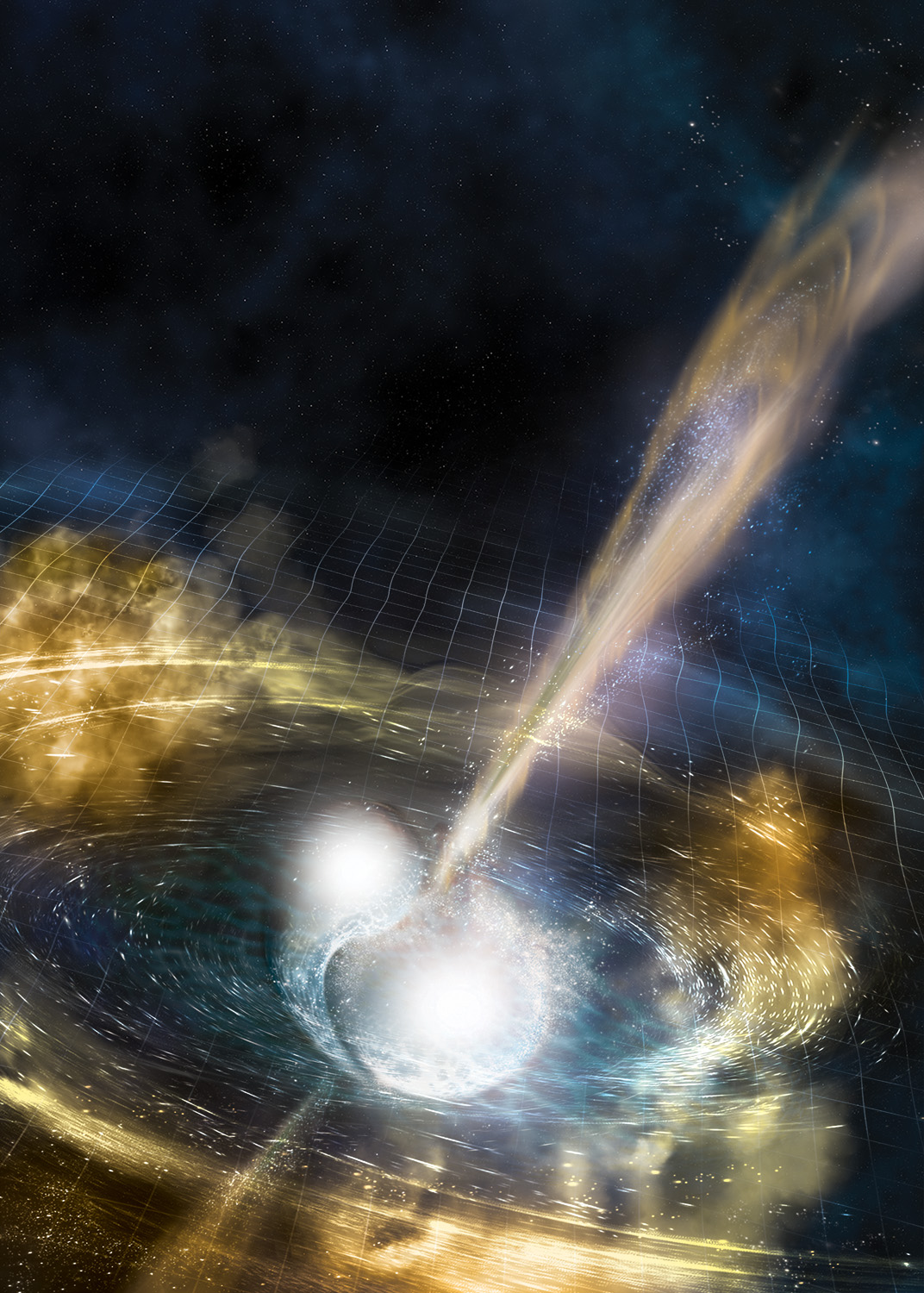
On August 17, 2017, the two detectors of Advanced LIGO, along with VIRGO, zeroed in on what appeared to be gravitational waves emanating from a pair of neutron stars spinning together – a long-held goal for the LIGO team. An alert went out to collaborators worldwide and within hours some 70 instruments turned their sights on the location a mere 130 million light-years away.
Their combined observations, spanning the electromagnetic spectrum, confirm some of what physicists had theorized about this type of event and also open up new areas of research. Thousands of scientists contributed to this accomplishment, including many at Stanford University, and published the initial findings Oct. 16 in Physical Review Letters and The Astrophysical Journal Letters.
“It’s a frighteningly disordered, energetic place out there in the universe and gravitational waves added a new dimension to looking at it,” said Robert Byer, professor of applied physics at Stanford, faculty at the Kavli Institute for Particle Astrophysics and Cosmology (KIPAC), and a member of LIGO who provided the laser for the initial detector. “For this event, that new dimension was complemented by the signals from the other electromagnetic wavelengths and all those together gave us a completely different view of what’s going on inside the neutron stars as they merged.”
This observation and the others that are likely to follow could help further the understanding of General Relativity, the origins of elements heavier than iron, the evolution of stars and black holes, relativistic jets that squirt from black holes and neutron stars, and the Hubble constant, which is the cosmological parameter which determines the expansion rate of the universe.
Stanford and LIGO
LIGO is led by the Massachusetts Institute of Technology and the California Institute of Technology, but Stanford was brought into the collaboration in 1988, largely due to the ultra-clean, stable lasers developed by Byer. The Byer lab developed the chip for the laser in the initial LIGO detector, which they installed in the early 2000s and lasted the lifetime of the initial LIGO project, which concluded in 2010. Lasers for the Advanced LIGO built upon Byer’s earlier work, an effort led by Benno Wilkie of the Albert Einstein Institute Hannover, a former postdoctoral scholar in Stanford’s Ginzton lab.
“We were looking for the problems that LIGO couldn’t actually worry about yet. We wanted to find those and solve them before they became roadblocks,” said Byer. “One thing that allowed Stanford to contribute to LIGO in these extraordinary ways is we have this long tradition of engineering and science working together – and that’s not common. Great credit also goes to our extraordinary graduate students who are the glue that hold it all together.”
Daniel DeBra, professor emeritus of aeronautics and astronautics, designed the original platform for LIGO, a nested system so stable that, in the LIGO detection band, it moves no more than an atom relative to the movement of Earth’s surface. Another crucial element of the vibration isolation system is the silicate bonding technique used to suspend LIGO’s mirrors. As a visiting scholar at Stanford, Sheila Rowan of the University of Glasgow adapted this technique from previous work at Stanford on the Gravity Probe B telescope.
The Dark Energy Camera (DECam), the instrument used by the Dark Energy Survey, was among the first cameras to see in optical light what the LIGO-VIRGO detectors observed in gravitational waves earlier that morning. DECam imaged the entire area within which the object was expected to be and helped confirm that the event was a unique object – and very likely the event LIGO had seen earlier that day.
Many people at Stanford and the SLAC National Accelerator Laboratory are part of the Dark Energy Survey team. Aaron Roodman, professor, KIPAC member and chair of particle physics and astrophysics at SLAC, developed, commissioned and continues to optimize the Active Optics System of DECam.
Looking to the future, DeBra and colleagues including Brian Lantz, a senior research scientist who leads the Engineering Test Facility for LIGO at Stanford, are improving signal detection of Advanced LIGO by damping the effects of vibrations on the optics.
Other faculty are improving the sensitivity of the Fermi Large Area Telescope (LAT), a instrument helmed by Peter Michelson, a professor of physics and KIPAC member, that can both confirm the existence of a binary neutron star system and rule out other possible sources. Its sister instrument on Fermi, the Gamma-Ray Burst Monitor, detected a gamma ray burst coming from the location given by LIGO and VIRGO 14 seconds after the gravitational wave signal.
LIGO is offline for scheduled upgrades for the next year, but many of the researchers are already working on LIGO Voyager, the third-generation of LIGO, which is anticipated to increase the sensitivity by a factor of 2 and would lead to an estimated 800 percent increase in event rate.
“This is only a beginning. There are many innovations to come and I don’t know where we’re going to be in 10 years, 20 years, 30 years,” said Michelson. “The window is open and there are going to be mind-blowing surprises. That, to me, is the most exciting.”
What’s so special about neutron stars
A neutron star results when the core of a large star collapses and the atoms get crushed. The protons and electrons squeeze together and the remaining star is about 95 percent neutrons. A tablespoon full of neutron stars weighs as much as Mt. Everest.
“Neutron stars have some of the strongest gravity you’ll find – black holes have the strongest – and thus they give us handles on studying strong-field gravity around them to see if it deviates at all from General Relativity,” said Mandeep Gill, the outreach coordinator at KIPAC and a member of the Dark Energy Survey collaboration.
Astronomers proposed the existence of neutron stars in 1934. They were first found in 1967, and then in 1975 a radio telescope observed the first instance of a binary neutron star system. From that discovery, Roger Blandford, professor of physics at Stanford and KIPAC member, and colleagues confirmed predictions of the General Theory of Relativity.
Blandford said the calculations related to the system Advanced LIGO saw are even more complicated because the stars are much closer together and could only be completed by a computer. This observation continues to support the General Theory of Relativity but Gill is hopeful that additional binary neutron star systems may begin to inform extension to the theory that could reveal how it fits with quantum theory, dark energy and dark matter.
“One of the things I find terribly exciting about these observations is that not only do they confirm aspects of astronomical and relativistic precepts but they actually teach us things about nuclear physics that we don’t properly understand,” said Blandford. “We certainly have many things that we’ve speculated about and thought about – and I have to believe that some of that will be right – but some of it will be much more interesting than what we could anticipate.”
As we observe more of these systems, which scientists anticipate, we may finally understand long-standing mysteries of neutron stars, like whether they have earthquakes on their crust or if, as suspected, they have small mountains that send out their own gravitational wave signal.
“Even though we’ve been doing astronomy since the dawn of civilization, every time we turn on new instruments, we learn new things about what’s going on in the universe,” said Lantz. “If the elements heavier than iron are actually made in events like this, that stuff is here on Earth and it’s likely that was generated by events like this. It gives you sort of a way to reach out and touch the stars.”
Blandford is also KIPAC Division Director in the Particle Physics and Astrophysics Directorate and professor of particle physics and astrophysics at SLAC; Byer is also a professor in SLAC’s Photon Science Directorate.
Additional Stanford contributors to the LIGO multi-messenger observation include Edgard Bonilla, Riccardo Bassiri, Elliot Bloom, David Burke, Robert Cameron, James Chiang, Carissa Cirelli, C.E. Cunha, Christopher Davis, Seth Digel, Mattia Di Mauro, Richard Dubois, Martin Fejer, Warren Focke, Thomas Glanzman, Daniel Gruen, Ashot Markosyan, Manuel Meyer, Igor Moskalenko, Nicola Omodei, Elena Orlando, Troy Porter, Anita Reimer, Olaf Reimer, Leon Rochester, Aaron Roodman, Eli Rykoff, Brett Shapiro, Rafe Schindler, Jana B. Thayer, John Gregg Thayer, Giacomo Vianello and Risa Wechsler.