Stem Cells and Neural Repair: A Q&A with KIBM's Mark Tuszynski
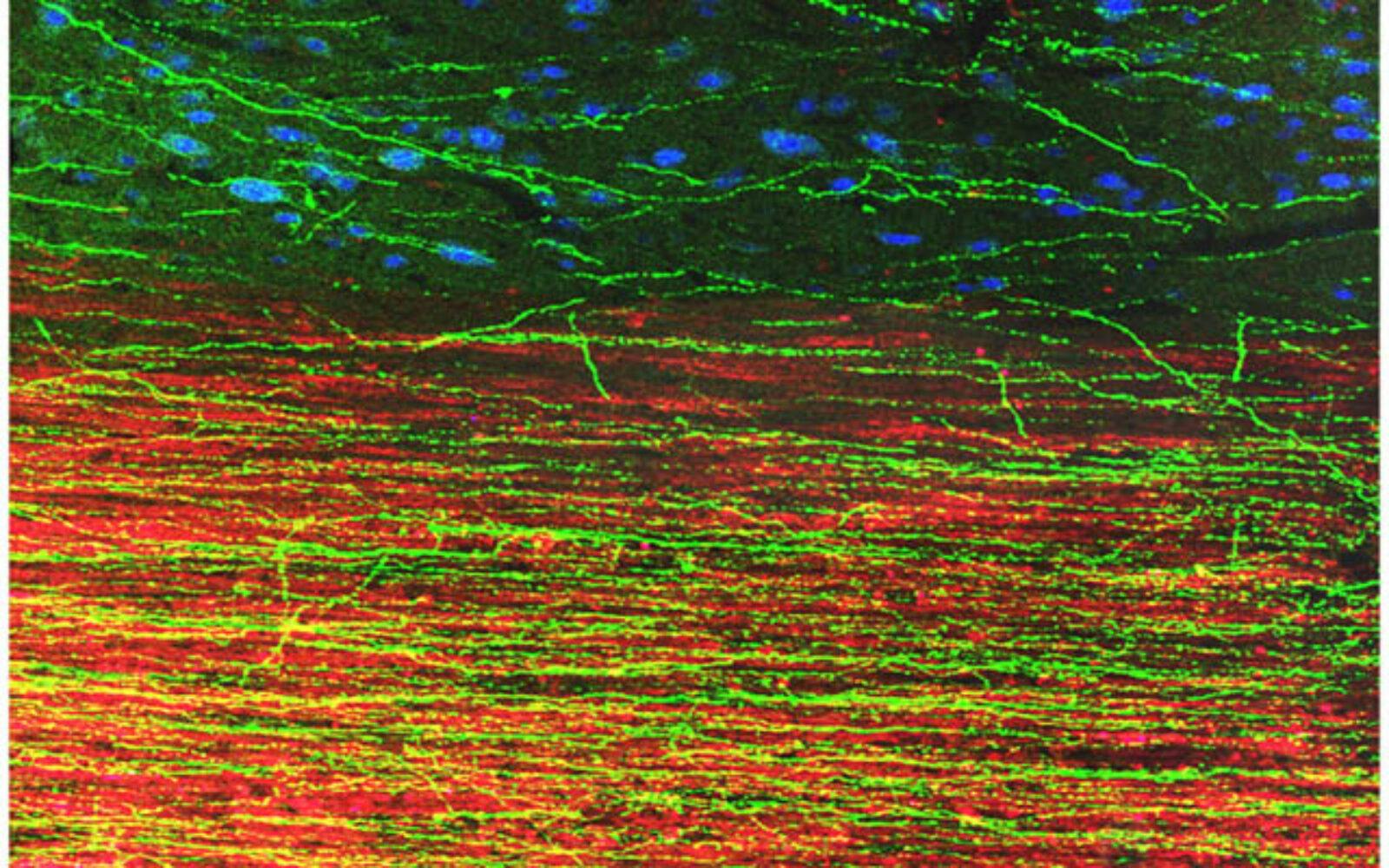
Neurons generated from human stem cells and implanted into rats with spinal cord injuries can grow long axons and form synapses, according to new research from UC San Diego. Researcher Mark Tuszynski discusses the findings and what they tell us about the nervous system’s ability to repair itself.
August 20, 2014

Stem cell therapies for spinal cord injury are already being tested in clinical trials but 95 percent of these trials fail. That’s partly because the damaged central nervous system prevents cells from regrowing. Researchers affiliated with the Kavli Institute of Brain and Mind at the University of San Diego, who are trying to optimize stem cell therapies for repair, have now shown that human neuronal stem cells grafted into rats with injured spinal cords can grown axons that extend very long distances and even form synapses with other neurons. (Read UCSD press release: Dramatic Growth of Grafted Stem Cells in Rat Spinal Cord Injuries) Furthermore, even neuronal stem cells generated from aged tissue—in this case the skin of a healthy elderly man—can do so.. The work, published in the online edition of the journal Neuron, raises the possibility that individuals with spinal cord injuries could one day be treated with stem cells derived from their own tissue. It also raises fundamental questions about how these cells manage to grow in such a hostile environment.
The Kavli Foundation asked senior author Mark Tuszynski, MD, PhD, professor of Neurosciences and director of the UC San Diego Center for Neural Repair, what his new study tells us about the intrinsic capacity of the nervous system to repair itself.
THE KAVLI FOUNDATION: What does this study teach us about the potential of the nervous system to repair itself?
Mark Tuszynski: It tells us that neurons from an early stage of development, placed into the fully adult and injured nervous system, still mount a remarkable ability to extend axons. It had been generally assumed that axons would not extend for long distances through the adult nervous system, and especially through the injured adult nervous system. These studies very clearly show that in fact early stage axons—even those generated from elderly humans—retain an impressive growth capacity.
TKF: Your results suggest that human neuronal stem cells have the intrinsic ability to overcome the inhibitory factors that prevent adult neurons from regrowing after spinal cord injury. Do you have any ideas what those might be?

TUSZYNSKI: Early stage neurons are expressing an internal genetic program that drives growth; this is likely a key factor allowing their extensive growth. Early stage neurons also are not meylinated, meaning they lack the protective sheath around their axons, and are not reacting to myelin-associated inhibitors in the adult nervous system. This too likely supports their extensive growth. In fact, we even have some early evidence that early stage neurons prefer to grow on myelin. Understanding the intrinsic growth program of early stage neurons and their interactions with myelin are two of the key questions that we now seek to better understand.
TKF: In this study, you implanted into adult rats neuronal stem cells that you had generated from skin cells taken from an elderly man. Were you surprised that these aged cells were able to regenerate?
TUSZYNSKI: We guessed that neural stem cells derived from induced pluripotent stem cells (iPSCs) generated from healthy adults would behave like neural stem cells obtained from embryonic stem cells, or neural progenitor cells of the developing spinal cord. So we aimed to challenge the cells further by using donor cells from an elderly human. Indeed, as we now know, these cells extended axons over the longest distances we have yet observed.
TKF: In a past study, you showed that rat neuronal stem cells could regenerate across an injury site, form connections, or synapses, with other neurons and even improve limb movement after damage to the spinal cord. But in this study, using human neuronal stem cells, you didn’t see any improvement in the rats’ ability to move. Why do you think that is?
TUSZYNSKI: First, these grafts developed rifts in their centers consisting of collagen that essentially separated the grafts into two compartments. Without communication across the gap, one would not expect functional improvement. We are working to eliminate these rifts. Interestingly, they do not form when we place grafts in larger animal models of spinal cord injury or in rats when we use a contusion model of injury (instead of an open transection lesion). Second, hand movement is a more complex function than simple locomotion, require integration of inputs from many sources. So it might be more difficult to detect improvement in hand function. Third, these human cells may have been maturing more slowly than the rat cells used in our previous study, and we might need to wait longer before functional improvement based on human cells occurs. Fourth, the human axons extending out of the injury site were not myelinated, and this might have prohibited functional recovery. These are all possibilities that we are testing.
TKF: What’s the next step in this research?
TUSZYNSKI: A very important step is to take the cells to longer time periods of survival, to determine the rate at which human cells are maturing. A second step is scaling up the technology to larger animal models. A third step is eliminating the collagenous rifts to allow relays to potentially form across the injury site.
From the basic neuroscience perspective, these cells in and of themselves are fascinating subjects of study that can teach us a great deal. Along these lines, we are examining the transcriptional state of the cells as they actively extend axons, and whether molecular switches for axon growth can be identified. If so, we might be able to turn on these switches in adult neurons to improve axon regeneration. There are many, many other basic and applied questions that emerge from this work.
— Lindsay Borthwick, Summer, 2014