Talking Qubits and Origami Machines
Research Highlights from Kavli Nanoscience Institutes
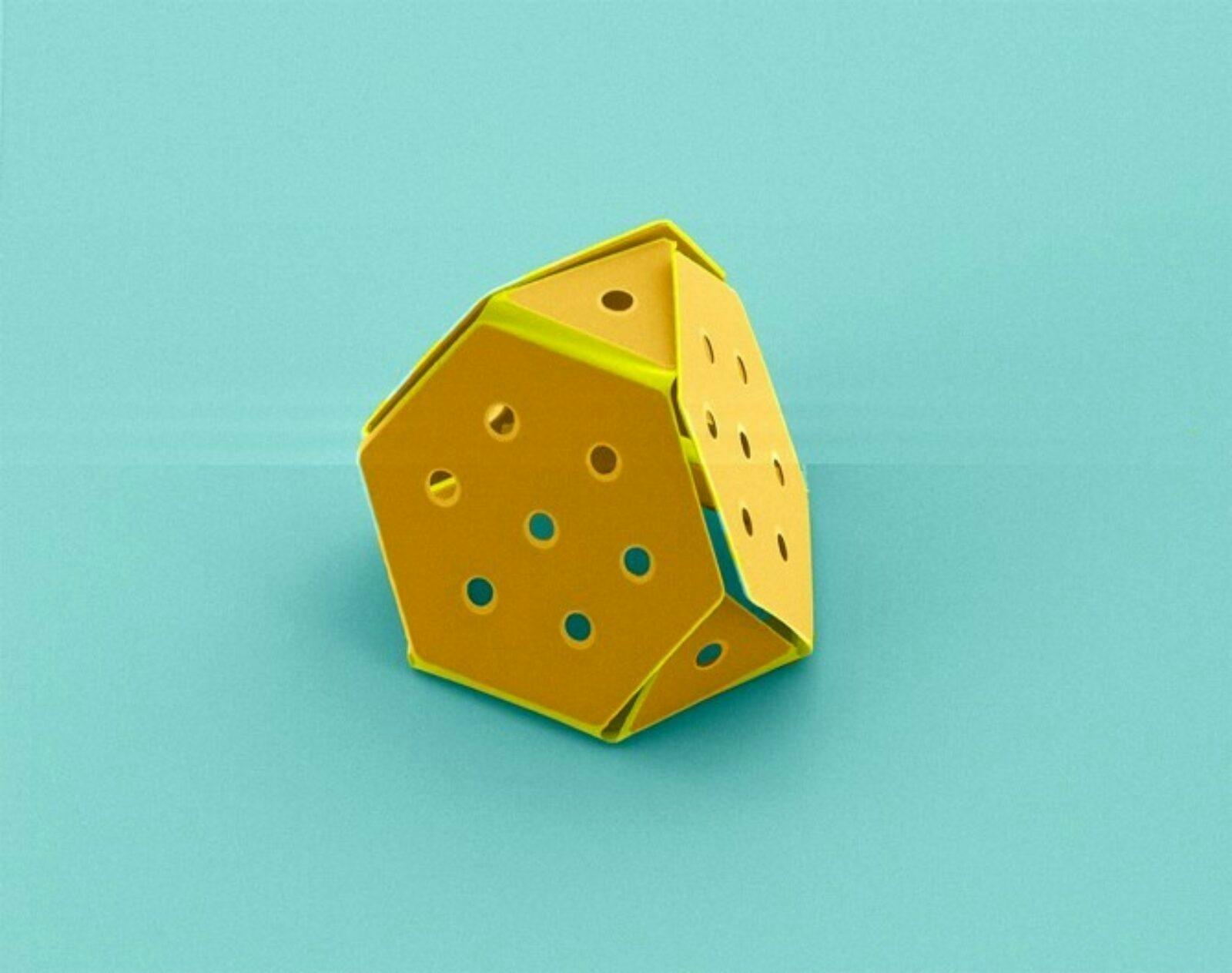
The ability of nanoscience to manipulate quantum states is on display at Kavli Nanoscience Institutes. At UC Berkeley, researchers found a way to make otherwise quick-to-vanish excitons last long enough to enable future discoveries. Meanwhile, at Delft Institute of Technology, researchers have developed different types of qubits to exchange information with one another, opening the door to quantum computers that combine different qubit technologies. And at Cornell, a team of researchers has taken a closer look at van der Waals superconductors with a tool that might provide insights into how these materials achieve superconductivity in disordered states. Also at Cornell, we witness the evolution of nanorobots from liquid-dwelling systems to machines that can move on dry land.
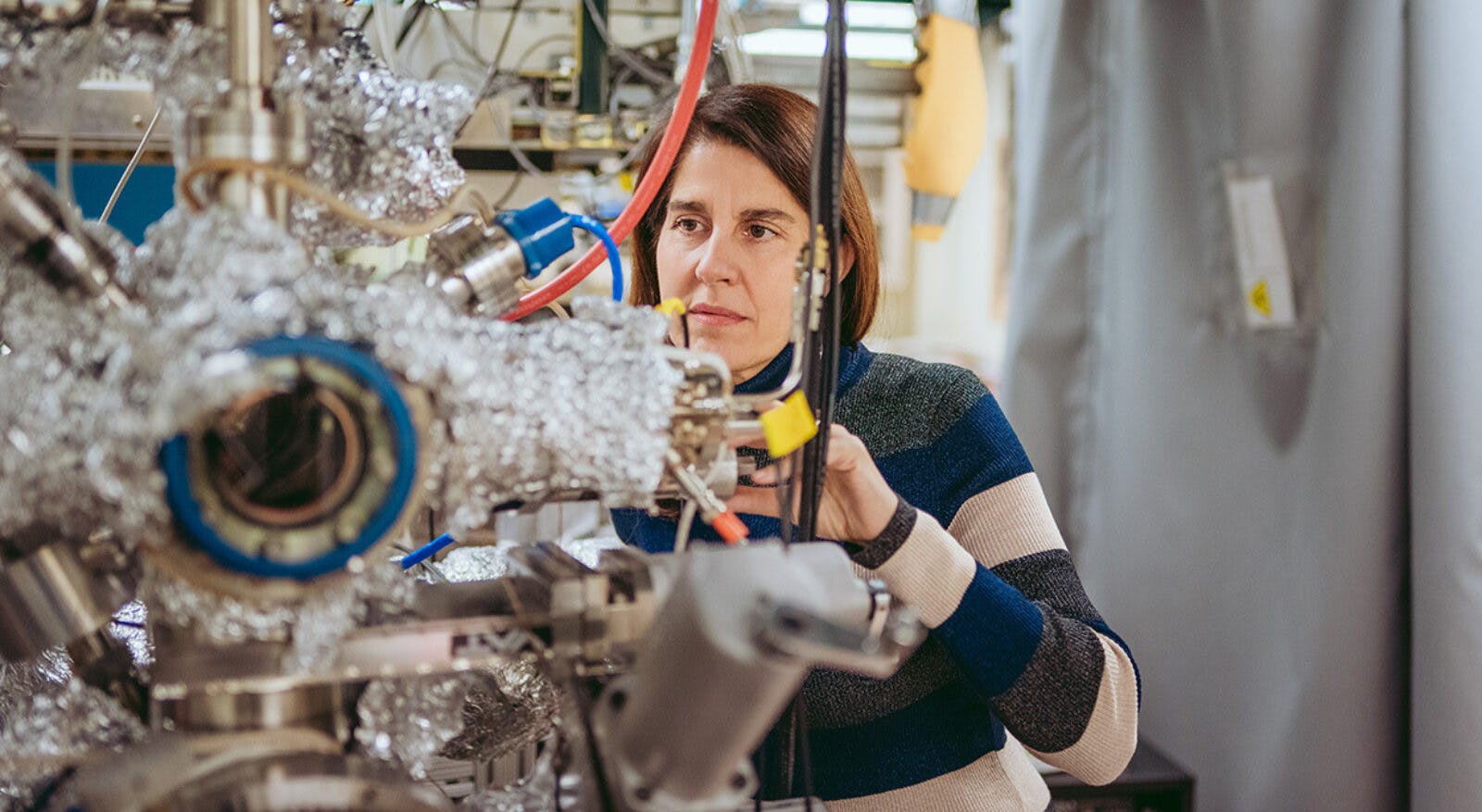
Excitons stay excited longer
Excitons occur when a semiconductor absorbs light, creating an excited electron coupled with a lower-energy electron vacancy (or hole). The exciton, alas, disappears when its two components reunite, which happens too quickly to use them to transfer information. Now, Alessandra Lanzara, a member of the Kavli Energy NanoScience Institute UC Berkeley, has found a way to make them last much longer. To do this, she coupled a state-of-the-art measurement technique that she helped pioneer -- time-spin-angle-resolved photoemission spectroscopy -- with a well-characterized topological insulator, bismuth telluride. The measurement device delivers short, intense pulses of light. When they hit the bismuth telluride, they produce excitons. After hundreds of hours of testing, her team found the right conditions to create an electron that rides on the material’s surface and a hole that resides below. These separated excitons last longer than quasiparticles whose components are closer to one another. The team then measured how these long-lasting excitons interacted with other charge carriers in the material and discovered that they maintained their strong spin polarization in the excitonic state. Lanzara believes this opens the door to future applications that take advantage of the exciton’s electronic, optical, and spin properties.
Talking qubits
There are many distinct types of qubits, the quantum states that hold information in quantum computers. They all have their strengths and weaknesses, ranging from instability and poor resolution to mass production difficulties. One obvious solution would be to use different types of qubits in applications best suited for them, such as semiconductor electron spins for processing and superconducting transmons for long-distance communication. Unfortunately, different qubit technologies do not ordinarily talk with one another. Now, Christian Andersen, a member of the Kavli Institute of Nanoscience at Delft and a member of QuTech, a quantum computing research hub, has found a way to change that. His team started by using a microwave signal to generate a fast-reacting Andreev spin semiconductor qubit. He then strongly coupled it with a superconducting transmon capable of transmitting information over long distances. While more work remains to be done, the scalability of Andreev qubits is similar to that of other semiconductor qubits, opening the door to quantum systems that can achieve new levels of performanceby optimizing the type of qubits they use for different tasks.

SQUID sees 2D superconductor secrets
Superconducting quantum interference devices, or SQUIDs, excel at resolving the behavior of miniscule magnetic fields at really low temperatures. That makes them a powerful tool for understanding van der Waals superconductors, which are held together by weak van der Waals forces rather than the molecular bonds that form rigid crystals in conventional superconductors. Their structure raises lots of questions about how these materials achieve superconductivity despite their relatively disordered molecules. Until now, researchers have been trying to answer those questions by using electric transport, which enables them to determine when the material transitions to a zero resistance (superconducting) state. Katja Nowack, a member of the Kavli Institute at Cornell, has found a way to measure another superconductivity property, the extent to which the material expels a magnetic field. This is another characteristic of superconductors. By measuring that property, Nowack can calculate how many electrons participate in the superconducting state. Her surprising conclusion is that van der Waals materials achieve superconductivity even though the samples are electronically disordered, raising even more questions about these fascinating materials.
Origami nanobots evolve to dry land
Nanorobots shaped by origami folds and powered by chemical reactions have come a long way. Yet nearly all of them operate only when submerged in water and at elevated temperatures. Even then, most are painfully slow. A new collaboration led by Kavli Institute at Cornell members Nicholas Abbott, Itai Cohen, Paul McEuen, and institute co-director David Muller have now found a way to enable nanorobots to move quickly on dry land at room temperature. The problem, as the researchers saw it, is that there are only a limited number of chemical reactions that can take place on the robot’s surface and cause the origami folds to bend and generate movement. So, instead of trying to find a new reaction pathway, the researchers looked closely at the steps already found in existing reactions. They found some occurred very rapidly and produced large motions. They essentially optimized the nanobots to use only those steps. The result is a system that takes only 600 milliseconds per actuation cycle. That’s fast enough to think about practical applications, such as systems that regulate flow based on the composition of a stream of chemicals.
