Tapping the Quantum Power of Large Objects
by Alan S. Brown
Simon Gröblacher explores the quantum secrets of systems containing billions of atoms, developing ways to link quantum computers together
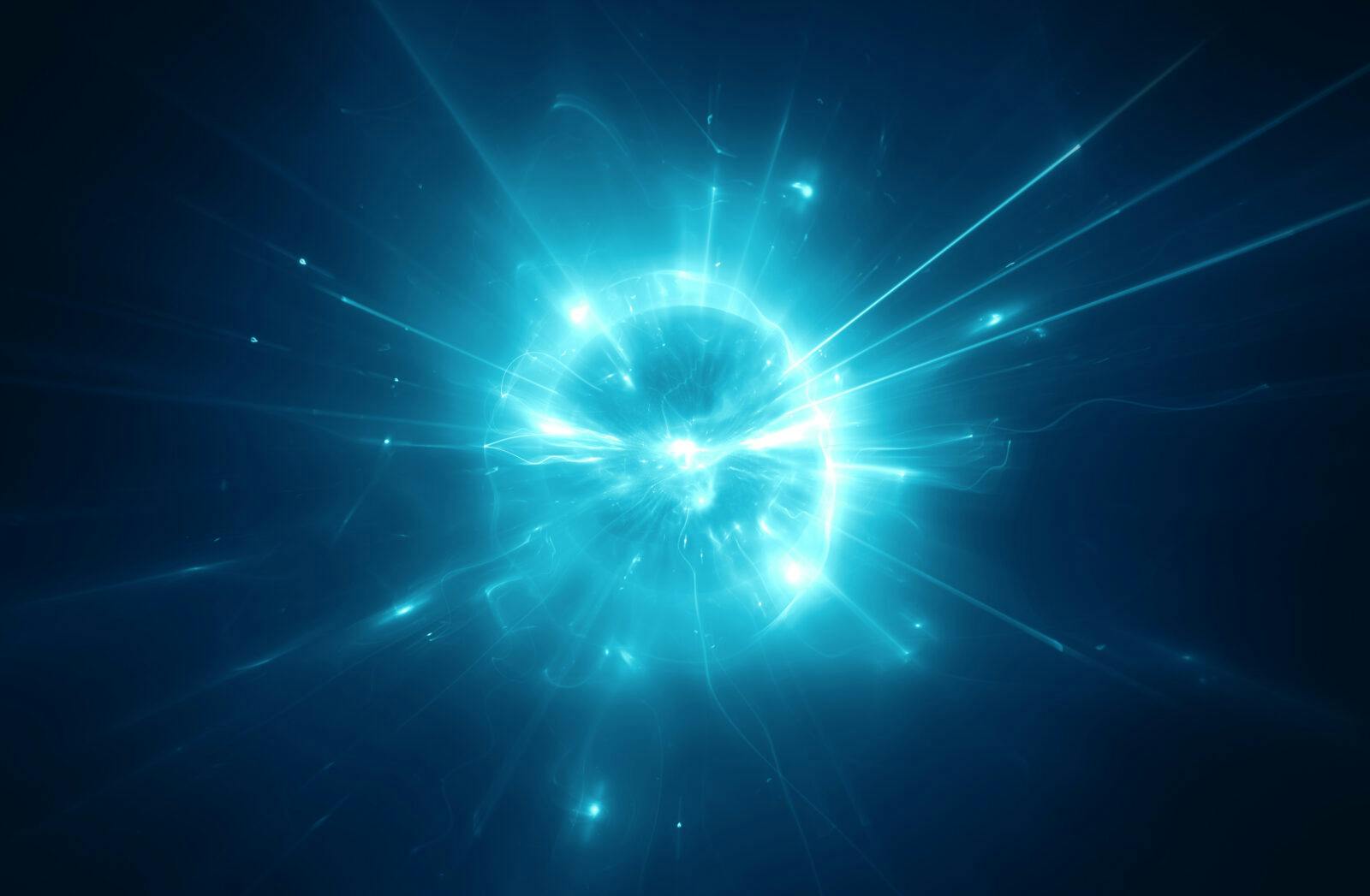
The Author
The Researcher
When scientists talk quantum mechanics, the discussion usually centers around individual subatomic entities like electrons, photons, bosons, and the like. The list rarely includes systems of billions of atoms—objects large enough to view under a good microscope.
Yet that is exactly the type of system that intrigues Simon Gröblacher, a quantum physicist and member of the Kavli Institute of Nanoscience at Delft University of Technology. His goal is to tap the quantum properties of what he calls “mechanical objects” to accomplish such practical tasks as networking quantum computers.
Gröblacher is well on his way to meeting that challenge. Last year, he demonstrated a quantum memory comprised of billions of atoms. It was able to interact with a single particle of light, a photon, take on its quantum state, and retain that state for several milliseconds. This may not sound like a long time, but quantum states typically collapse in nanoseconds or less, even in systems chilled to near absolute zero to slow the transfer of energy that makes them vanish.
This memory system will form the heart of a quantum repeater, a device that could store and resend quantum states (and the information they contain) before they wink out of existence. Repeaters could play a vital role in linking together thousands of quantum computers to tackle difficult mathematical problems.
This year, Gröblacher took another giant step towards that vision. He created a device that converts the quantum state of one type of photon into the quantum state of another—without either photon coming into contact with the other.
It does that by using his mechanical system as a go-between. By controlling the device’s structure, Gröblacher can coax the system to generate photons whose wavelengths allow them to rush along existing optical telecommunications networks without losing the information they hold.
These devices, Gröblacher said, are not only potentially practical devices but ways to advance the limits of quantum science.
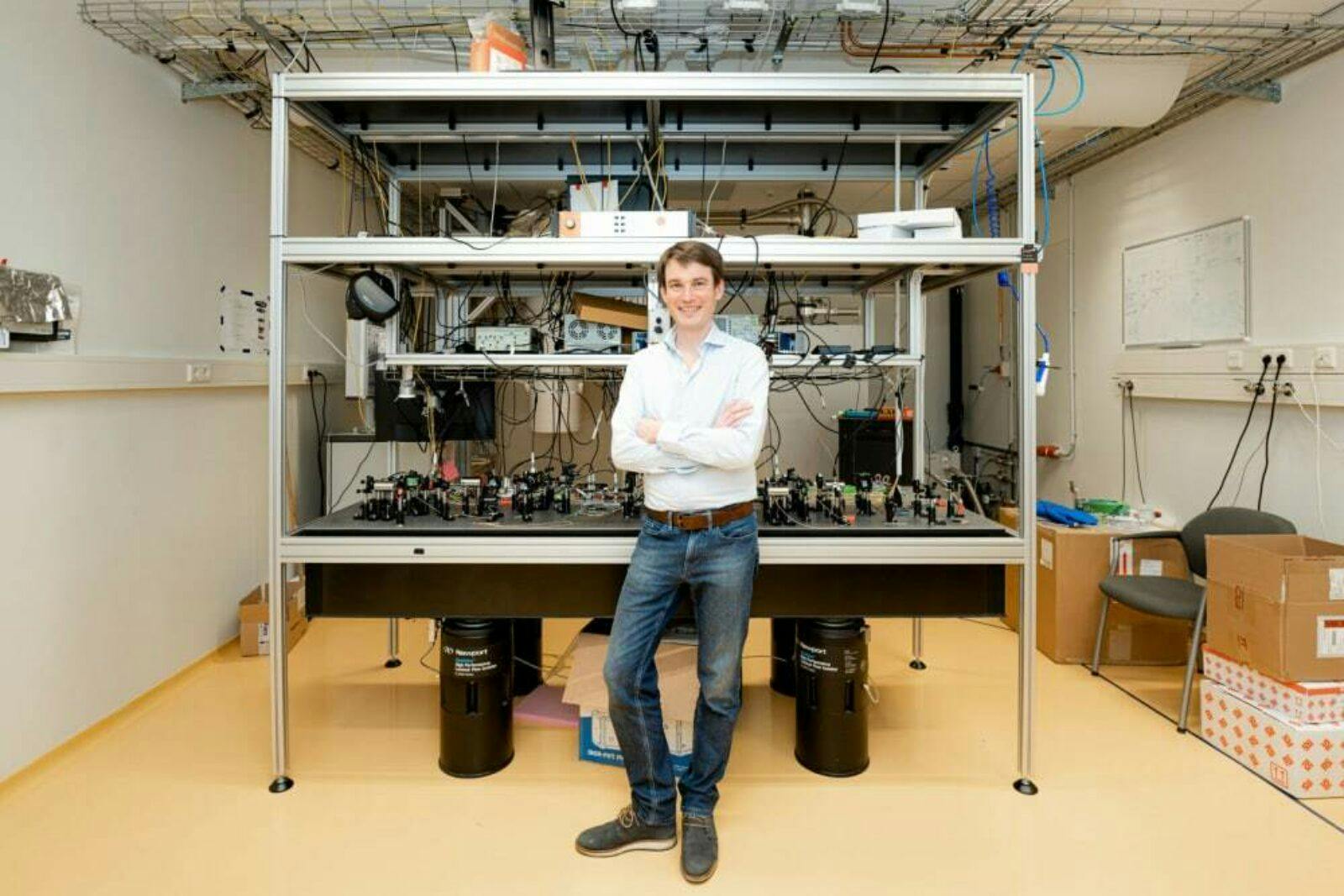
On the Edge
Gröblacher never intended to study quantum objects. “I know very few physicists who said when they were young, ‘I want to be a physicist,’” he said. “After high school, I was completely undecided. I tried astronomy, but I couldn’t personally measure things or grasp them because they were so far away.”
Paradoxically, his practical nature led him to quantum physics, the most theoretical of sciences: “I went from the largest things in the universe to the smallest,” he said. “Even as a young student in a small group of researchers, I found I could pose a question that was very profound, put together an optics experiment, and actually learn something new about quantum physics. It was very satisfying and limited only by my imagination.”
Gröblacher earned his Ph.D. at University of Vienna, followed by a three-year post-doctoral at California Institute of Technology with Oskar Painter, a member of the Kavli Nanoscience Institute. There, he continued to study optomechanics, how light interacts with mechanical systems. He joined Delft in 2014, where he continued to probe the quantum nature of larger and larger objects.
“Supposedly, anything can be described by quantum theory,” Gröblacher said. “Even many-body objects made up of billions of atoms should behave according to the rules of quantum physics. By linking with these large physical objects, we’re trying to push the limits of the science. We want to see how massive we can make an object and still observe quantum effects. According to quantum theory, there is no limit to size. Yet, in the reality of classic physics, that is not the case. So, what’s going on? Where’s the transition? How does it happen?”
This research places Gröblacher on the edge of quantum physics research. Yet the answers he discovers could yield practical benefits. Photons, often the object of quantum research, are hard to manipulate and couple with other types of systems. Large objects, on the other hand, can interact with other quantum systems as well as classical systems.
“If you can control them well enough, you could think about bridging different types of quantum systems that would ordinarily not talk to each other,” he said.
Resonators
Gröblacher’s resonators allow him to do just that, though it took a series of advances to get there. To understand them, consider a classical mechanical resonator. It is a system that oscillates at many frequencies, but some more strongly than others. A church bell is a good example, and so is a leaf quivering in the wind.
Quantum resonators are different. In the quantum world, properties are discrete; that is, they only exist in certain, specific energy states. There is no continuous path from one state to another, so they do not vibrate at all frequencies. Instead, energy jumps from one energy state to the next, as if it were crossing a river by jumping from stone to stone.
Like its conventional counterpart, a quantum resonator starts with many energy states. Reduce its temperature, however, and it will have less energy available to jump from state to state. As temperature approaches absolute zero, it will sink to its lowest possible energy state.
“At that point, the mathematical description of the billions of atoms in this resonator is exactly the same as for a single photon or an electron,” Gröblacher said. “Those billions of atoms can now be in a quantum state, like a superposition state, which is like having two energy states at the same time.”
This is where the magic happens. Since those billions of atoms are now behaving like a single quantum object, they can now interact with a photon of light on a quantum level. This entangles the two, so that they share the same quantum state even when they are physically separated.
Gröblacher is using the resonator for experiments involving quantum mechanical excitations, discrete packets of vibrational energy that make up an oscillation. His ultimate goal is to enable these excitations to travel across a waveguide, much the way electrons travel along a circuit in a semiconductor chip.
The resonator lies at the heart of the quantum memory system Gröblacher devised to store quantum states (which we can think of as information) for prolonged periods of time. In the wild, quantum states are fragile and degrade in far less than a millionth of a second. To use their information, researchers have found ways to preserve those quantum states for microseconds and, more recently, milliseconds.
Gröblacher believes his high-quality resonator structures could preserve those states for up to one to two seconds, an eternity in quantum mechanics, and to read out the data he has stored. This is long enough to use resonator memory to build a quantum repeater capable of storing and resending quantum information along a network.
Connecting
This yields a device that can “teleport,” or transport, the quantum state (or information) of a photon that has no mass onto a massive mechanical system made of billions of atoms, where he can store it and then read it out again.
To build a repeater, however, Gröblacher must be able to retransmit the quantum state he has stored in his memory. This can be done optically. Gröblacher, however, is more excited by his ability to use the resonator memory to convert the quantum state of one type of photon into that of another type of photon.
In one case, a microwave photon creates an entangled state with the resonator. The resonator then emits an optical photon that carries that same state as the original microwave photon. The system can also work the other way, starting with an optical photon and producing a microwave photon.
Gröblacher founded a company, QphoX, to transform that technology into what he calls the world’s first quantum modem to link quantum computers to one another. This would enable the creation of networks linking many thousands or tens of thousands of quantum computers, vastly expanding the type of complex problems they could solve.
Like a conventional modem, Gröblacher’s device will be bidirectional, so each networked computer can send and receive quantum data from any other computer. While the modem will operate near absolute zero, the photons it generates will share data over conventional, room-temperature optical networks. This eliminates the need for a cryochilled network while keeping costs manageable.
This past May, QphoX received a €2 million initial investment to commercialize the modem.
It may take several years to create a system that is reliable and cost-effective for commercial use. In the meantime, Gröblacher plans to stay busy in his lab, asking fundamental questions about the quantum universe and doing experiments to answer them.
It’s every kid’s dream, to do what he or she likes and get paid for it. It’s really fun, and I want it to stay fun by doing exciting science.
Simon Gröblacher