Termite Wisdom and New Materials
by Alan S. Brown
Research highlights from Kavli Nanoscience Institutes
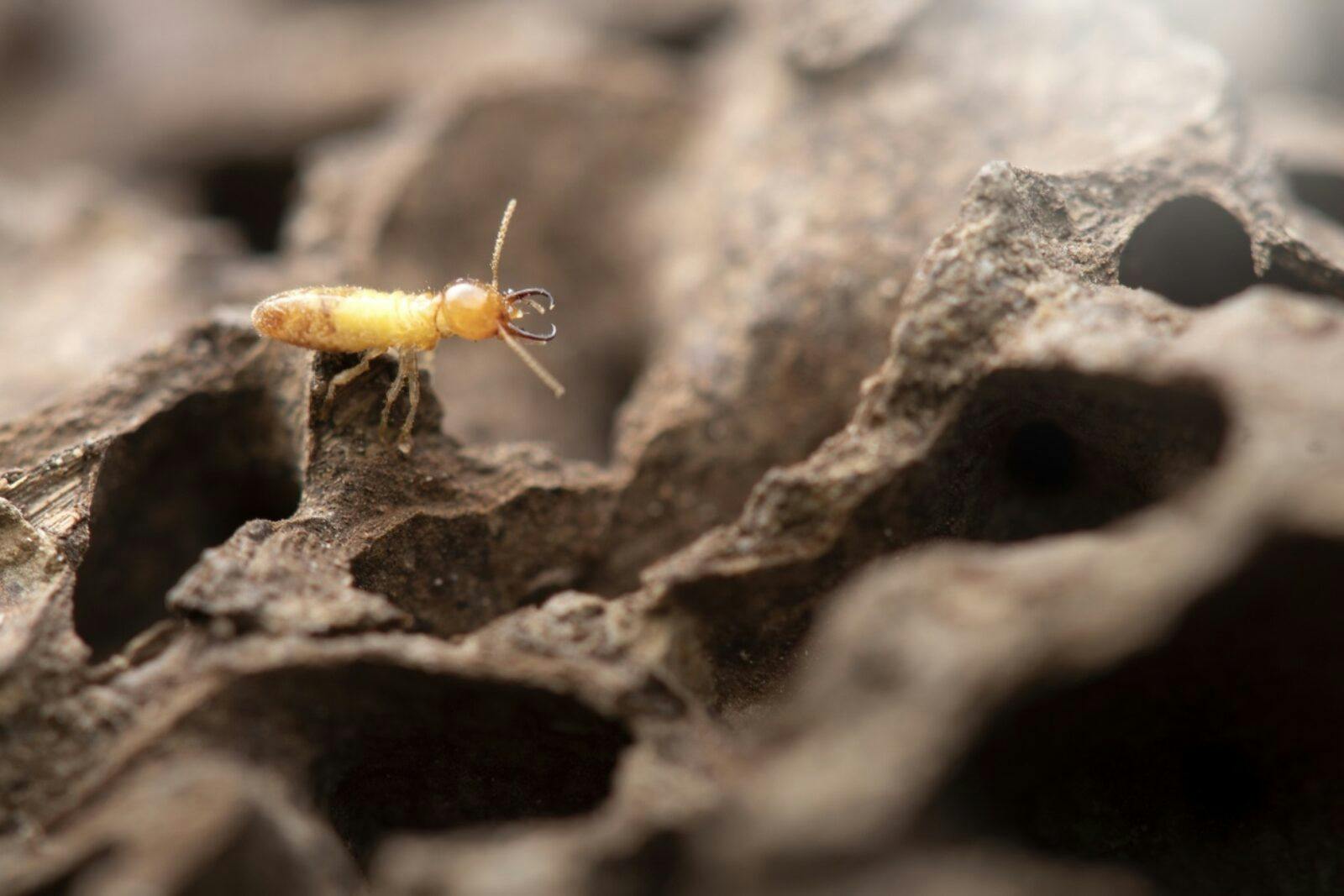
The Author
Scientists at Kavli Nanoscience Institutes have recently shared fascinating results and developed new tools with the potential to enable yet more discoveries. Take for example, efforts to learn to build with disordered materials (like the bubble structure in sponges and bread) in order to create engineering marvels like 4-meter-high termite nests optimized for stability and ventilation. Or the design of systems allowing for untethered solar-powered robots smaller than an ant’s head. There is also the computational and experimental discovery of a new type of material that could be used in solar cells, sensors, and non-volatile computer memory. And new superconducting nano-devices disclose photon phenomena that have not been previously observed, which can be used as a quantum amplifier to make a more sensitive detector for dark energy.
Termite wisdom generates new materials.
Only a few millimeters long, termites routinely build nests of sand, dust, dirt, saliva, and dung four meters high--the equivalent of two Empire State Buildings tall for humans. Slice nest open and you will see the interconnected asymmetrical structures like those that characterize sponges or bread. These networks may look arbitrary, but they actually optimize nest stability and ventilation. In fact, many natural structures, from bones and seashells to plant stems, use similar asymmetrical networks to achieve their unmatched mechanical properties. Now, Chiara Daraio, a member of the Kavli Nanoscience Institute at Caltech, has begun an ambitious program to deconstruct termite nest construction to develop rules that will let her use disordered networks to optimize strength, stiffness, durability, or any other material property. Daraio reasoned that termites used simple rules and limited resources to build. So, she created a software model to emulate their behavior. She then analyzes the disordered structures the model builds to discover the underlying rules behind them. This, she hopes, will one day become a new framework for materials and structural design.
Microrobots cast off the strings.
Until now, microscopically small robots were like marionettes: they needed string-like wires (or beams of light or magnetic fields) to power or control their motion. Now, members of the Kavli Institute at Cornell for Nanoscale Science have created a robot smaller than an ant’s head that walks autonomously without any type of external control. The research was led by Itai Cohen, Alyosha Molnar, Paul McEuen, and postdoctoral researcher Michael Reynolds. The robot’s brain uses a 1,000-transistor CMOS semiconductor clock circuit whose signals control the gain of the robot. A small solar cell powers both the CMOS processor and the robot’s platinum-based leg actuators. An outside vendor fabricated the processors on an eight-inch silicon wafer. The researchers then used a 13-step process to define the robot’s body, coat its legs with platinum, and remove the tiny robots from the wafer. CMOS circuitry and leg actuators that required only the scantest amount of power were critical to the project’s success. Improved microrobots could one day track bacteria, identify toxic chemicals, destroy pollutants, conduct microsurgery, and even remove plaque from arteries.
A simpler, less expensive solar cell.
Halide perovskites are a hot topic in solar cell research. The materials, which share a common perovskite crystal structure, show outstanding efficiencies of more than 25 percent in converting light into electrical energy. They are also inexpensive to produce, since they synthesized in solution and painted, sprayed, rolled, or spun onto substrates and heated to about 300 degrees F to form a solid surface. Then comes the hard part: researchers must add additional layers, one doped with positive ions and the other with negative ions, to ensure that electrons flow from one side of the device to the other. Now, a team of researchers co-led by Peidong Yang, director of the Kavli Energy NanoScience Institute at UC Berkeley, have demonstrated a ferroelectric halide perovskite with a bandgap compatible with visible light absorption, whose polar structure creates an electric field without any doping. This could reduce costs still further. Also, the cesium germanium tribromide material is free of lead and the health concerns it raises. The experimental fabrication and demonstration of this material was guided by computational methods to identify the composition within a vast set of possible materials.
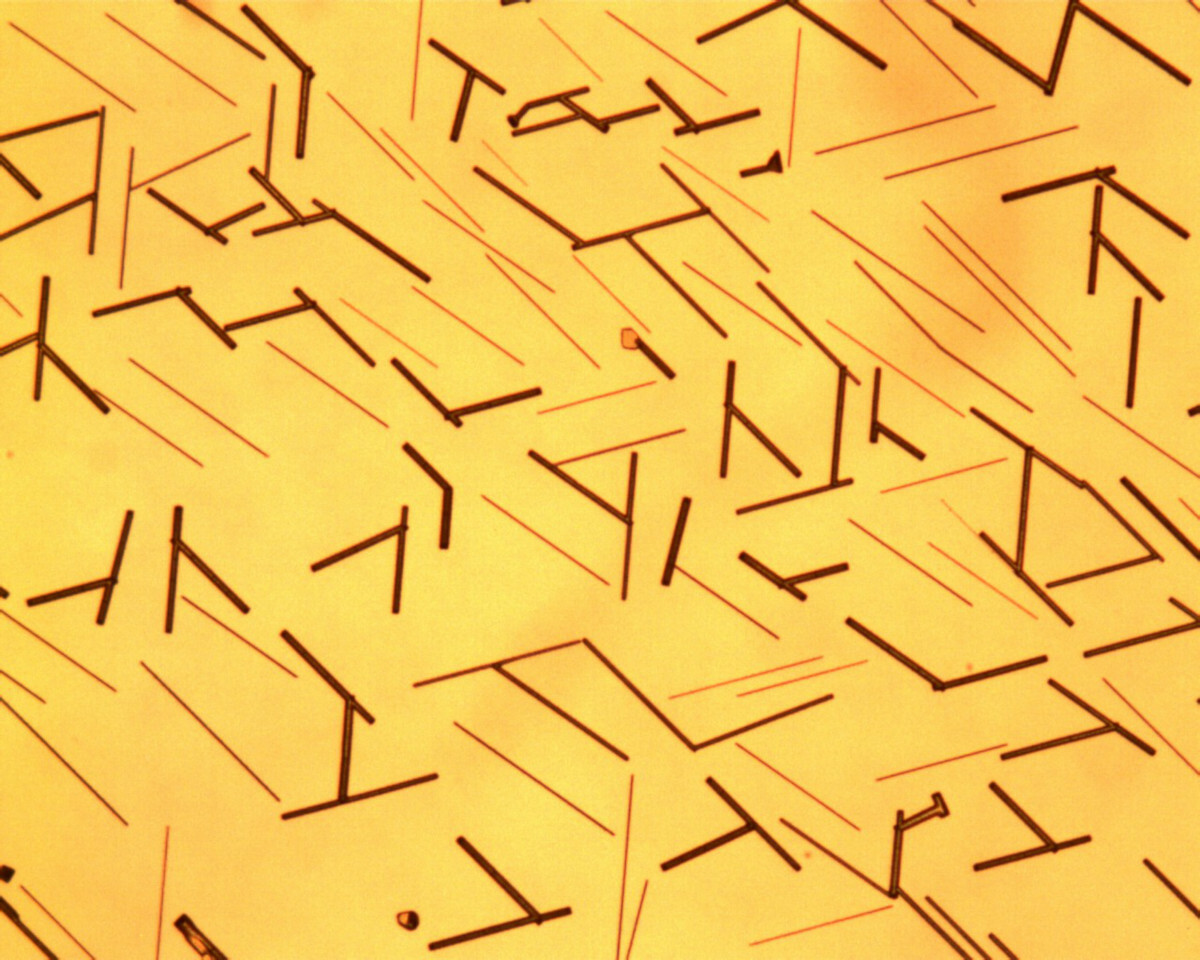
Quantum chip gives light a squeeze.
A new type of photonic chip generates and measures quantum states in light with high enough quality for quantum information processing. In fact, it could replace the bulky experimental setups now needed to perform the job. The key, according to senior investigator Alireza Marandi, a member of the Kavli Nanoscience Institute at Caltech, is the ability of the lithium niobate chip to squeeze the complex amplitude of light on one side of the chip and measure it on the other. This reduces noise at the quantum level, making it easier to separate signal from noise in order to measure and process the light’s resulting state. Engineers use squeezed states to boost the sensitivity of LIGO, the observatory that uses laser beams to detect gravitational waves. This provides a way to access similar technology on a chip and also provides a potential route for photonic circuits for optical computing.
Quantum amp works like heat pump.
Researchers have built a quantum heat pump from particles of light (photons) that could enable us to hunt for dark matter with increased accuracy. The key breakthrough is the device’s ability to transfer photons two ways. To understand how this works, consider what happens when you put a six-pack in icy cooler. The heat flows from the warm beer cans to the cold ice, which causes some ice to melt as they reach an equilibrium temperature. But heat pumps make the system flow in the opposite direction, capturing heat from winter air to warm a home. In the quantum world, researchers began with a photon pressure circuit cooled to a few millidegrees above absolute zero. This sounds cold, but some photons retain more thermal energy than others. Coupling these excited photons to higher frequency cold photons cools them to their quantum ground state. Their latest work takes this a step further, using an extra signal to pump photons preferentially in one direction. This cools one part of the circuit even lower, making its photons more sensitive to the radio frequency waves emitted by dark matter. The research was done by a collaborative team that included Gary Steele, a member of the Kavli Institute of Nanoscience at Delft University of Technology.