The Age of Silicon’s Supporting Cast
by Alan S. Brown
Huili Grace Xing wants to take compound semiconductors a big step further
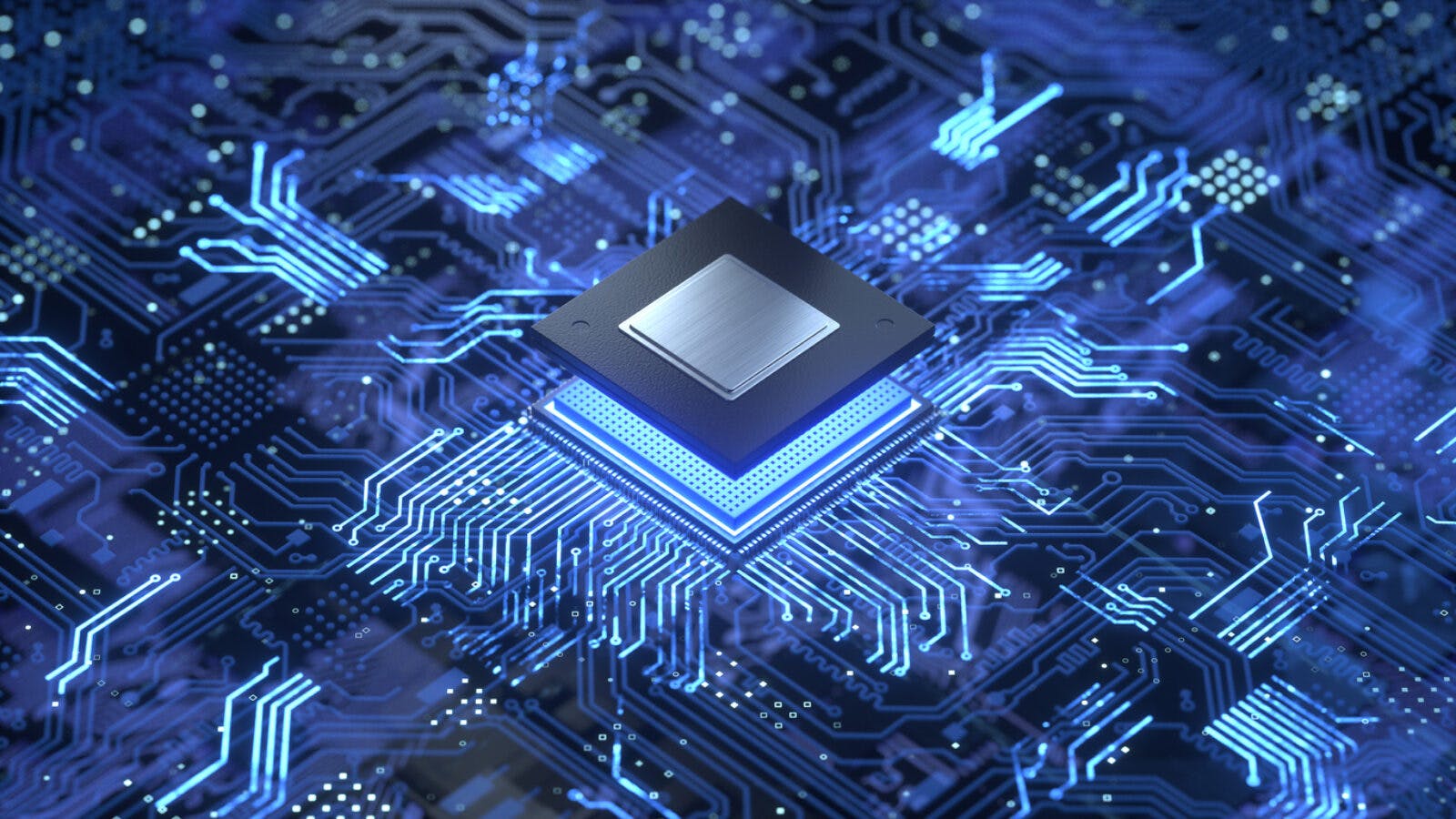
The Author
The Researcher
We live in the Age of Silicon, the ubiquitous semiconductor found in everything from smartphones and computers to automobiles and surgical robots.
Yet silicon cannot do everything. It cannot convert digital data and transfer it over the internet’s optical networks. It cannot display vibrant colors on phone and television screens or capture high frequency 5G wireless signals on a smartphone.
That takes compound semiconductors, materials made of two or more elements (like aluminum nitride or indium gallium arsenide). Although they are much harder to create than silicon, they excel at tasks silicon manages poorly.
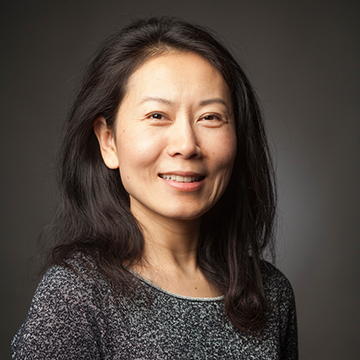
Huili Grace Xing, a member of the Kavli Institute at Cornell for Nanoscale Science, has spent a career taming compound semiconductors. She is a world-renowned expert in gallium nitride, which is used in cellular base stations and energy-saving LED lights. Today, the lab she runs jointly with her husband, Debdeep Jena, is pushing the boundaries of what compound semiconductors can do.
One example is an alloy of gallium oxide and aluminum oxide that can handle extremely high amounts of electrical power. Placing just a few square millionths of a meter on a semiconductor might one day make it possible to eliminate the need for transformers, the type used to recharge smartphones and power televisions, Xing said. It might also alter the economics of transformers that manage power on the electrical grid.
Such possibilities are heady. They are certainly a long way from Xing’s childhood in Wugang, a small city in China’s Henan Province, where the “information highway” sounded like a mystery she wanted to solve.
Down to the Details
Xing was always curious and liked to get into the nuts and bolts of how things worked. Her parents, both doctors, nurtured this trait and suggested she study physics at Beijing University, a top research school, to find answers to all her questions.
By the time she started college in the early 1990s, Xing’s curiosity had turned to the nascent “information highway,” a metaphor people used to describe the then-new concept of moving information over the internet.
“It was the most exciting thing I had heard of,” she said. “I could physically see highways, concrete, bridges, high-speed trains, and other technologies with my eyes. But the idea of an information highway was very mysterious to my generation.”
“Physics taught me the fundamentals, and that was, and remains important,” Xing said. “But the answers were too general. I was more interested in getting answers to my specific questions about how the internet’s components worked, so I switched to applied physics.”
She discovered that although silicon microchips did the computations, it took a lot of supporting devices to convert electronic data into light, route it to its destination along a tangle of optical fibers, and demodulate it and transform it into electrons once it reached its destination. Many of those devices were based on compound semiconductors.
Xing dived in. After graduation, she continued her studies at Lehigh University in Pennsylvania. The school had excellent materials laboratories, and Xing liked the values behind American scientific research.
“The United States attracts the best talent from around the world,” she said. “It has a very encouraging and mature system that values innovation, hard work, and integrity. That was very attractive to me.”
When Xing arrived at Lehigh in 1996, the winner of the race on to create energy-efficient blue and green LEDs just emerged: gallium nitride. Zinc selenide was another contender. Yet, no matter how perfect researchers grew their crystals, the selenide crystals degraded quickly when used. As Xing began to unravel this mystery, she wanted to build devices based on the materials she was studying.
That led her to University of California, Santa Barbara, for her Ph.D. There, she pivoted to work on gallium nitride, another compound semiconductor that—not surprisingly—combined limitless potential with defects that kept it from broader use. Her advisor, Umesh Mishra, played a key role in the systematic studies of gallium nitride that led to the first radio-frequency amplifiers, while other faculty members had pioneered the development of high-efficiency blue and green LEDs and lasers.
A Partnership
Xing also met her future husband, Debdeep Jena, in Mishra’s lab.
“We were pursuing slightly different research projects, but we had fun discussing physics and semiconductor devices and what was going on in the field,” Xing recalled. “It was a very, very exciting time. Little did we realize that what we were studying back then would lead to big changes in today’s technology.”
Xing pauses, then laughs. “I guess our love story was more or less like a research project,” she said. “When you start a research project, it’s definitely love at first sight. You think, ‘That’s interesting, I’m interested.’
“Then you explore a little bit and there are problems, and you say, ‘I don’t know if I can overcome these issues.’ But if you’re still interested, you keep exploring until, hey, you solve the problem.”
They solved that problem by marrying. After graduation, they moved to the University of Notre Dame, where they set up separate research laboratories but collaborated extensively.
There have been many great collaborators in science, Xing continued. Some stuck for a lifetime, others ended as bitter enemies.
“That’s an indicator of just how crazy the dynamics can be,” Xing said. “For us, though, it’s different because we’re married. If we disagree, we cannot just go our separate ways. Sometimes, we have to walk away and cool down temporarily, but we believe our partnership like a religion. It’s not something we are going to give up on just because we have a hard time, because other times, it’s very, very good.”
That family dynamic extends through the large research group Xing and Jena jointly run today. At Cornell, it includes a third principal investigator, three research associates, five post-docs, 25 graduate students, and a sprinkling of undergraduate researchers. Yet Xing thinks of them as extended family, especially in terms of the time she spends with her students.
“When they become independent researchers, they might become our competitors,” she said. “But will be a healthy competition that is what moves our knowledge and our research field forward. We all want future generations to do better, and we are our student’s biggest cheerleaders.”
Oxides
At Notre Dame, Xing continued to investigate gallium nitride and its sister semiconductor aluminum nitride. Unlike silicon, a single element that is relatively simple to turn into a perfect crystal, compound semiconductors like gallium nitride are hard to build without defects in their crystalline structure. Much of Xing’s time was spent finding ways to reduce those defects or find a way around them so she could build devices, such as high-power ultraviolet LEDs to disinfect water and high-voltage transistors to power conversion units.
She joined Cornell in 2015 and continued to work on gallium nitride power diodes (which allow electricity to flow in only one direction) that could handle thousands of volts. Then, as gallium nitride moved into the mainstream, she turned her focus to aluminum nitride as well as oxides, another large family of semiconductors whose properties range from insulating to superconducting.
She concentrated on gallium oxide, a material with a very wide energy bandgap. A material’s bandgap is the amount of energy needed to pop an electron free of its atom so it can zip through a material and conduct electricity. The greater the bandgap, the higher the electric field a semiconductor material can handle without breaking down.
Gallium oxide’s bandgap is so large, scientists once classified it as an insulator. More recently, they developed ways to manipulate those crystalline structures—gallium oxide has several of them—to create large-bandgap semiconductors. This makes gallium oxide a promising material for switching and converting electrical power.
Most researchers focused on a type of crystal called beta-gallium oxide. It has a wide bandgap and good stability (always a must when running lots of energy through something). It is also relatively easy to grow.
Xing and Jena wanted to go further. They were intrigued by beta’s cousin, alpha-gallium oxide. It has an even larger energy bandgap than beta, but it is less stable and harder to grow.
Perhaps, they thought, they could improve alpha’s properties by blending it with another material. They chose alpha-aluminum oxide (better known as sapphire, which also has a relatively large bandgap.
The good news was that sapphire’s crystalline structure closely resembles that of alpha-gallium oxide, though it is not an exact match.
To get around that problem, Riena Jinno, a visiting Ph.D. student on a fellowship in the Jena-Xing lab, turned to a technique called molecular beam epitaxy (MBE). It evaporated gallium and aluminum atoms and mixed them with oxygen. As they gently settled on the sapphire template, where they took on the structure of the underlying foundation.
“It’s like building a Lego house,” Xing said. “We want the atoms to descend so gently that the atomic interactions snap those atomic ‘Legos’ into the right place.”
Getting that structure right is extremely difficult. Not only are atoms so small, but the material growers had to manipulate three different elements, gallium, aluminum, and oxygen. That is much harder than building a crystal from one or two elements. Over time, though, the Jena-Xing team developed “recipes” involving time and pressure that let it build stable alpha-aluminum gallium oxide samples with large bandgaps.
A surprising future
“We definitely hope we can commercialize this technology,” she said. “Materials like aluminum nitride and gallium oxide, these materials can sustain very large voltages—hundreds or even thousands of volts—across only a tenth of the diameter of human hair. That means we can make the circuitry we use much, much smaller.”
How small? Today, smartphones, laptops, television sets, and many other electronic devices come with transformers to convert AC current into the direct current needed to operate or recharge these devices. Very-high-bandgap materials might enable manufacturers to build those converters into the device itself. Users could lose the transformer and just plug an electrical cord into an outlet.
Arrays of very-large-bandgap chips might let us build much smaller and easier to install transformers for the electrical grid. This could lower the cost of transformers used to transmit solar and wind power and to build the infrastructure needed to support widescale electric vehicle recharging.
Recently, the Jena-Xing lab created a rare phenomenon previously thought impossible: the co-existence of superconductivity and quantum Hall effect in superconductors. The quantum Hall effect, a phenomena involving voltage moving through a thin layer of material, only occurs in a strong magnetic field. Superconductors conduct electricity without any resistance, but cannot typically exist in a strong magnetic field. “Magnetism is like kryptonite for superconductivity,” Jena noted.
Yet Jena, with Xing’s help, managed to marry the two. Working with collaborators at the Naval Research Laboratory (NRL) and Qorvo, the researchers grew highly perfect layers of gallium nitride and niobium nitride atop one another. The flawless arrangement of the atoms let the researchers use a smaller magnetic field to induce the quantum Hall effect in one material without disrupting the superconductivity found in the other.
Xing, who helped develop some of the materials, says the research may provide “a path forward for us to truly integrate different modalities of quantum technologies — computation, memory, communication.”
“In our lab, the collaboration on any given project is ultimately driven by one person, the one who is passionate about the research, and helped by the other,” she said. “We contribute in different ways. These problems are typically complex and there are several possible paths we could take, but we still have to pick a path together.”
Xing has come a long way since she started investigating compound semiconductors more than two decades ago. Yet her passion and curiosity have not changed.
“I love the material systems that we study,” she said. “While we always keep in mind how conventional materials work, we want whatever we study to offer something unique, something that builds on what we already know.”
In other words, another piece of the complex infrastructure that makes the Age of Silicon possible.