The Brains of Electric Fish
by Sally Johnson
Theoretical neuroscience explores the functions and mechanisms of neural circuits across species
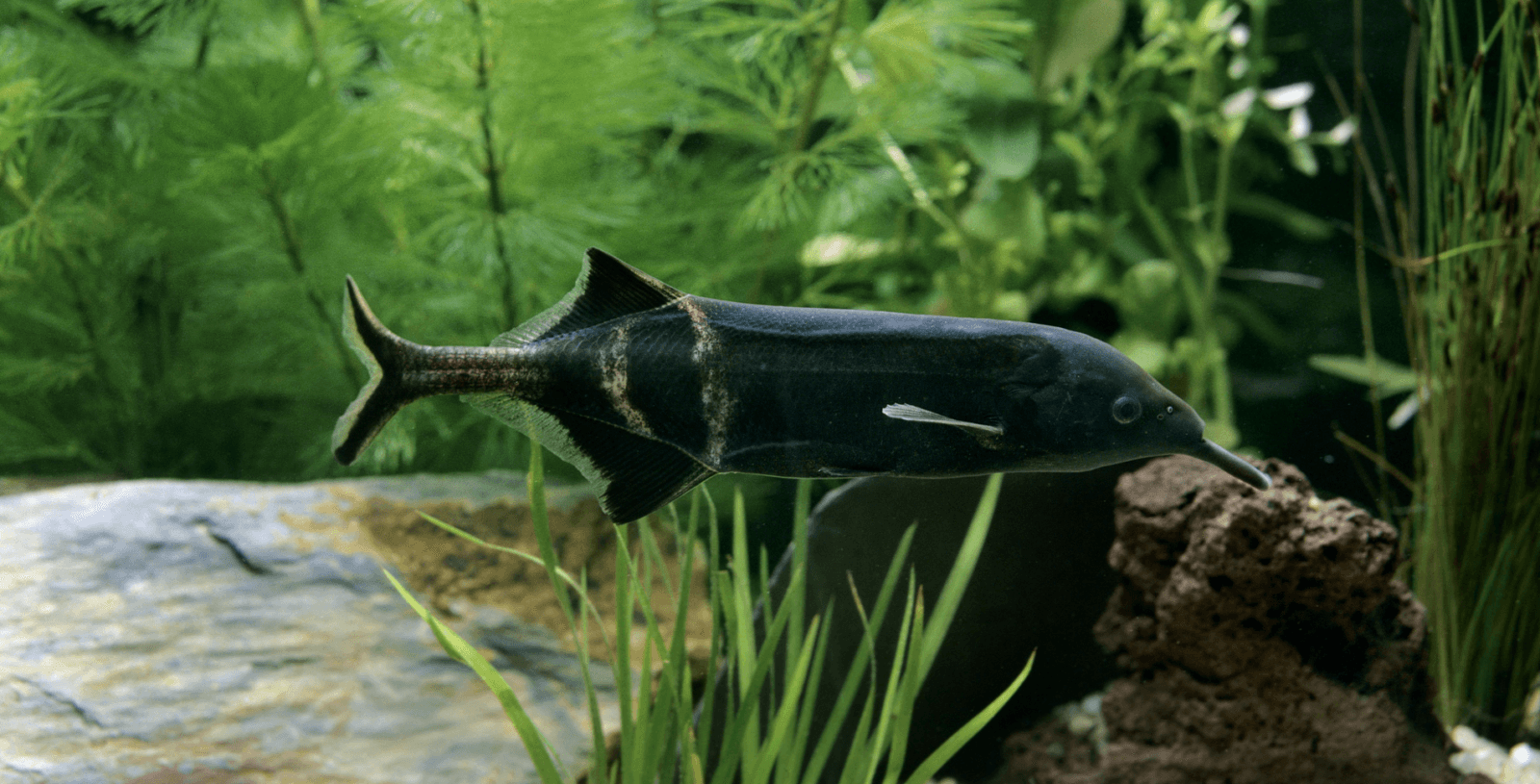
The Author
The Researcher
Theoretical neuroscience “ranges all the way from extracting information from data to building models to try to figure out what’s going on within the brain,” says Larry Abbott, co-director of the Kavli Institute for Brain Science. “Broadly, it’s applying computational and mathematical methods to neuroscience data at all levels and building models of how you think cognition takes place—and everything in between."
Falling in Love with Neuroscience

Abbott’s story about how he got into the field of theoretical neuroscience is a good one. He was a professor of particle physics at Brandeis University in 1989, when someone suggested he go visit well-known neuroscientist Eve Marder’s lab to check out the work going on there. Abbott didn’t know Marder well yet, but walked over out of curiosity.
“I was definitely looking around for things to do because particle physics was entering into kind of a quiet time after a great revolution,” recalls Abbott. “Eve wasn’t there, but her postdoc showed me the experiments and neurons and I just instantly knew I was going to switch. It was like falling in love at first sight.”
He credits Marder with tutoring him for at least a year, and then they went on to work together for many years. “She really opened the door for me,” Abbott says. “Through her generosity, it was like going back to graduate school as a professor. I gradually learned the field, and I give a lot of credit to Eve because I really knew nothing.”
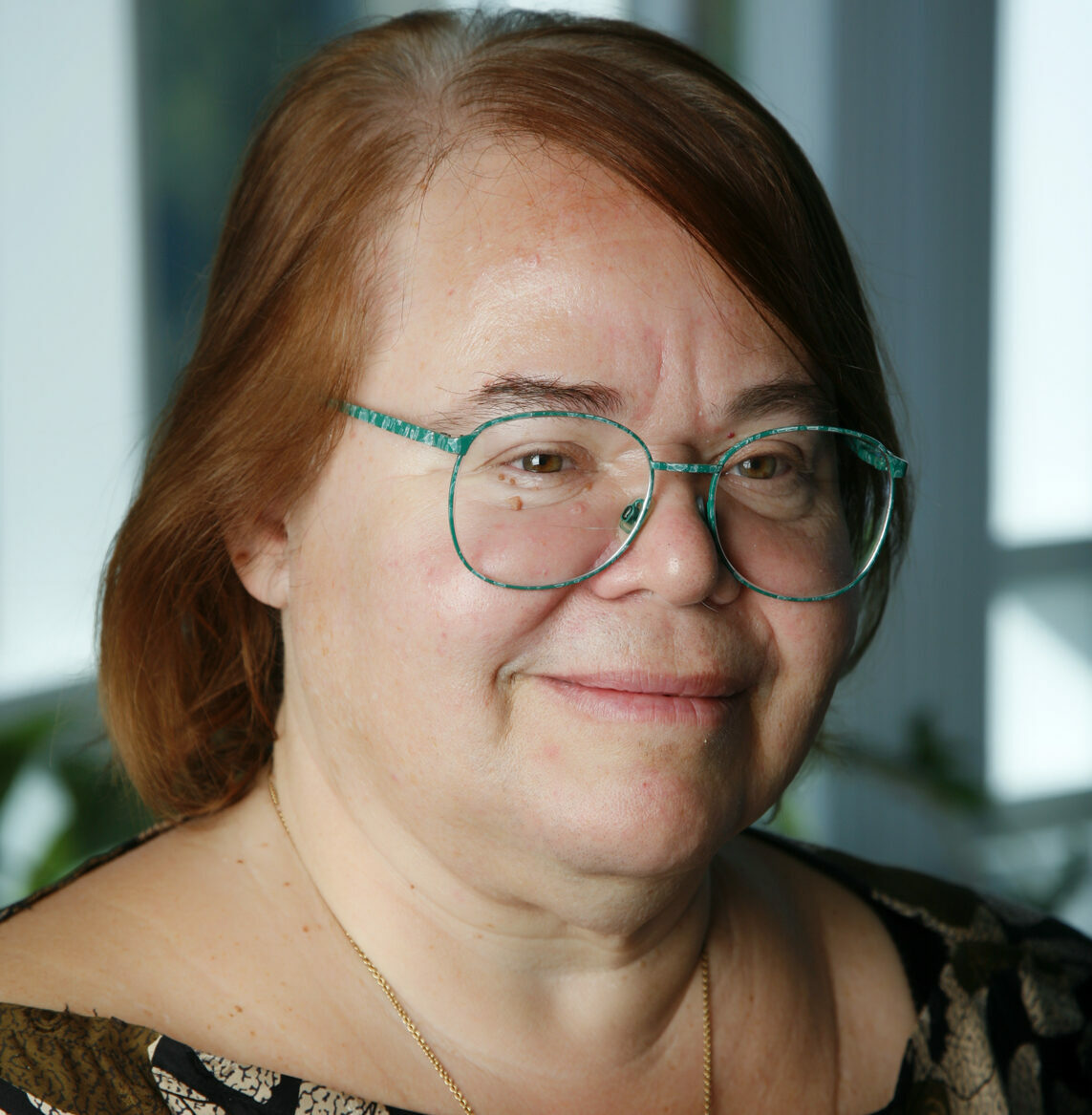
The two developed what’s known as the “dynamic clamp method” in 1993 to allow experimenters to simulate mathematically modeled conductances (the degree to which an object conducts electricity) into live neurons to see their effect on circuit output.
Today, Abbott especially enjoys looking at neural circuits with some sort of specialized function and exploring how they work. His research involves “math on a board,” as well as computer programs that he and his students write to simulate these specialized functions.
One recent example of Abbott’s work on a neural circuit with a specialized function is a project with Nathaniel Sawtell, an associate professor of neuroscience at Columbia, and graduate student Salomon Muller on electric fish, which can detect very tiny electric fields given off by the prey they’re interested in.
Electric Fish
“Electric fish also generate a strong electric field of their own for communication and sensing,” he explains. “It’s a little like if you’re running and your head is bouncing around. You need to see things not as if someone’s jiggling a camera, so your body adjusts for your motion and you can figure out what’s going on in the world. It’s a similar system—the electric fish essentially has to learn to do this because the field it produces is modified as a function of any water changes or if it grows or gets injured.”
Learning is interesting in neuroscience because, when it occurs, you can watch a neural circuit change. “Nate Sawtell, Salomon Muller, and I were able to make some nice advances in understanding how this circuit works in electric fish,” Abbott says.
It’s always surprising when the math actually works out, Abbott emphasizes. “It’s like a miracle when you do all that math, then the experiment, and you realize you got the same number,” he says. “These miracles don’t happen often in neuroscience, but it occasionally happens. The real miracle is that abstract thinking, when applied correctly, can actually work.”
In more recent work on the electric fish, the Columbia group got all the way down to the cellular level. “When you work on a circuit, you first look broadly at its function, then start getting down into the individual neurons, and even the individual cells to explore how they’re able to do what they do,” Abbott notes. “In this case, we could go that whole distance and put it together into a coherent picture, which was really exciting.”
Abbott has also done work on flies in the same way, focusing on how flies remember properties of odor and how they navigate.
All of this work is collaborative, one of the aspects Abbott enjoys most about theoretical neuroscience. “These projects are intense collaborations with the people who do the experiments,” he adds. “The key to success is the ability to collaborate across the lab-theory boundary, and communication makes it all work.”
Being a theoretical neuroscientist means you can jump around and explore different areas. If you build a lab, you can’t one day decide to do mice, then move onto flies the next day, or another creature the next day “unless you’re a theorist,” points out Abbott. “It’s important that we do that, because we carry ideas from one area to another. I work on flies, fish, and also olfaction in mice and on motor systems—a lot of different topics.”
And as experiments get more complicated and data gets bigger, the need for theoretical neuroscience is growing. Another thing Abbott works on is the connectome, and electron microscopy is allowing researchers to see basically every connection within a fly brain. As you can imagine, it’s a gigantic data set. “It’s difficult to think about big data like that without the tools and the ability to try out your ideas within models that theory brings,” he says.
Neuroscience technology is “unbelievable now,” Abbott adds. “It’s soared ahead with optical and recording methods, and now the connectome. We’re all trying to catch up to the data flood. How do we best intellectually process it to pull out the broad ideas? That’s where we are now and it’s really due to the incredible technology that’s been developed. It’s truly amazing what we can do now.”