The Immense Power, Beauty, and Promise of Multi-Messenger Astronomy
by Adam Hadhazy
Q&A with Daniel Holz from the Kavli Institute for Cosmological Physics
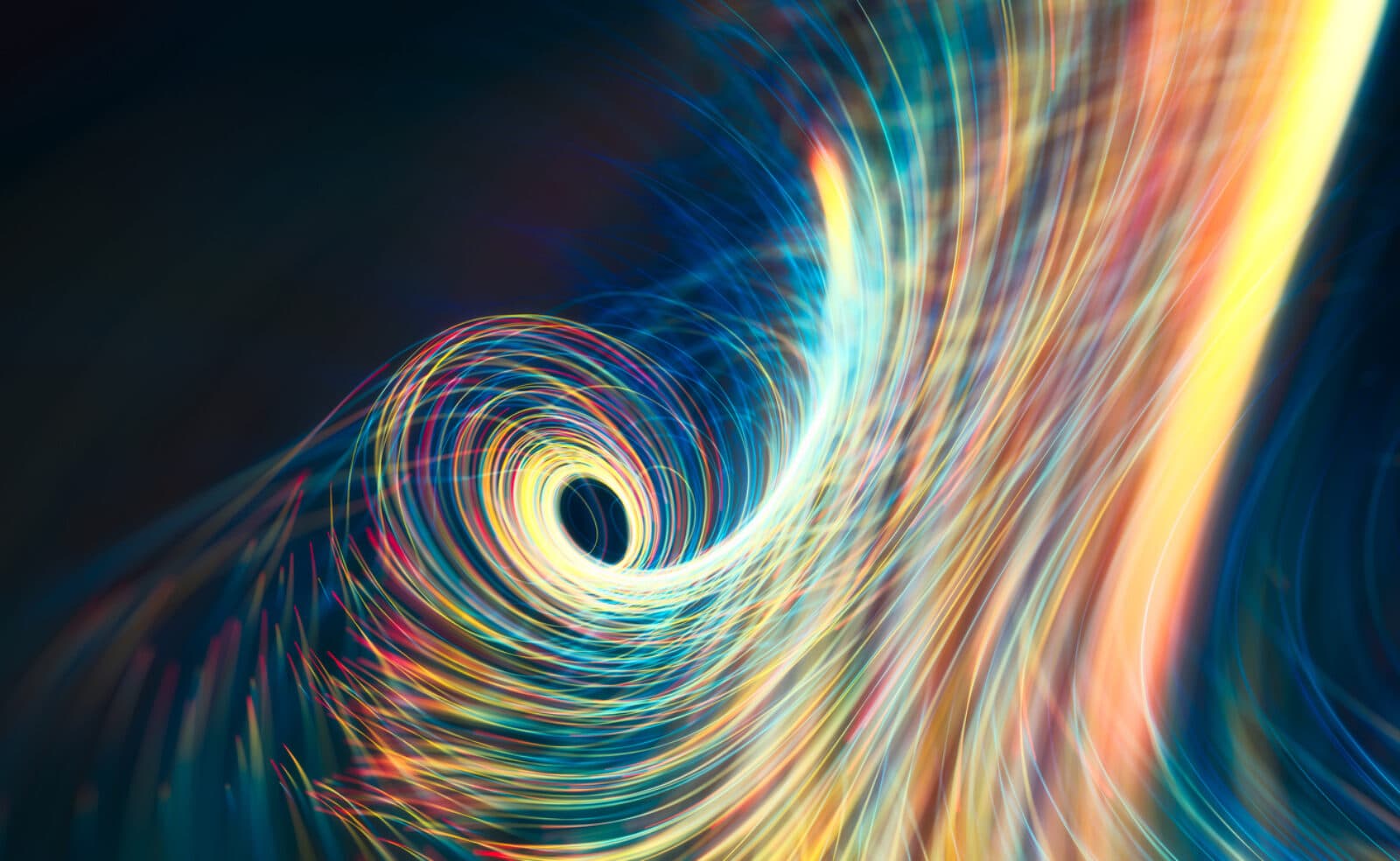
The Author
Astronomy ain't what it used to be . . . and that is a great thing. For most of its history as a science, astronomy rested solely upon light visible to the human eye. That all changed in the 20th century when scientists learned to capture invisible kinds of light, ranging from radio waves to gamma rays, as well as atomic nuclei—dubbed cosmic rays—and ghostly particles called neutrinos raining down from space. In 2015, scientists detected yet another kind of cosmic messenger: the ripples in spacetime known as gravitational waves.
Thanks to the development of ever-more sensitive instruments, these four kinds of cosmic messengers are now routinely garnered. A new field known as multi-messenger astronomy has accordingly emerged, combining observations of the same astrophysical event to glean novel insights into the universe's workings.
To learn more about the promise of multi-messenger astronomy, The Kavli Foundation spoke with Daniel Holz, a professor at the Kavli Institute for Cosmological Physics (KICP) at the University of Chicago. Holz works on the frontlines of multi-messenger astronomy and is a member of the international collaboration for LIGO (Laser Interferometer Gravitational-wave Observatory), the groundbreaking facility that first detected gravitational waves.
How does multi-messenger astronomy build upon conventional, light-based astronomy?
Daniel Holz: In the past we've mostly just looked up and out at the universe. We've used all sorts of different telescopes, ranging from familiar optical telescopes like Galileo's very first one more than four centuries ago and the Hubble Space Telescope of today, to more exotic telescopes such as microwave ones—which "see" the same sort of light that heats up food in your microwave oven—and radio telescopes and x-ray telescopes. These telescopes have become very powerful, and we've learned all sorts of amazing things.
But now we also have the possibility of "listening" to the universe through gravitational waves, which are ripples in space and time arising from gravity. This is an entirely new way to learn things. We can also detect particles such as neutrinos and cosmic rays from distant sources, and this is sort of like the sources reaching out and touching us.
The most exciting discoveries come from combining these different senses. It's a much deeper culinary experience if you can taste your food and also see your food and smell your food.
The four kinds of messengers in multi-messenger astronomy—light, cosmic rays, neutrinos, and gravitational waves—each correspondingly interact with the matter in our instruments through the four fundamental forces of nature. Light interacts with matter through the electromagnetic force, for instance, while cosmic rays interact with matter through the strong force by colliding into atoms in our atmosphere just like in a particle accelerator. Neutrinos, meanwhile, interact with matter through the weak force, and gravitational waves of course interact with matter through gravity. Why is this important?
Because of the different properties of the forces underlying the messengers, each one probes a unique physical aspect of the sources. By combining more messengers, we develop a deeper picture.
For example, by looking at an astrophysical source, we get a sense of what's going on at its surface. By listening to the source in gravitational waves, we get a sense of what's going inside and around the source. The combination of the two is uniquely powerful in unveiling distant astronomical phenomena.
Can you describe how your research involves multi-messenger astronomy?
I've been helping to develop the brand-new field of gravitational-wave astronomy. One of the things I'm most excited to do is measure the expansion history of the universe. To do this, we use what we call gravitational-wave standard sirens, where we capture both light and gravitational waves from a source. The light tells us the size of the universe, and the gravitational waves tell us the age of the universe, and by combining these we learn about its evolution history. This gives us insight into the age of the universe through the Hubble constant, which is a measure of the recessional rate of galaxies as related to their distance. It also gives us insight into the amounts of dark matter and dark energy in the universe, the nature of gravity, and many other profound questions.
We've applied this method to the event designated GW170817, which was the gravitational-wave detection of two neutron stars crashing into each other in 2017. As part of the LIGO team, we worked extensively on many aspects of the discovery. We also identified an optical counterpart. Our group contributed to the discovery using the DECam on the Dark Energy Survey telescope in Chile. By combining the sound—the gravitational waves—and the light—from radio, through optical, to gamma-rays—the GW170817 discovery was truly revolutionary in many different ways, including providing insights into where all the gold and platinum in the universe comes from via neutron star collisions, and providing a fundamental measurement of the speed of gravity, which we found to be the same as the speed of light to at least 15 decimal places. My favorite result from GW170817 was that we used it as a standard siren to measure the Hubble constant.
All of these discoveries are multi-messenger; they were only possible through the combination of gravitational-wave and electromagnetic observations.
What is the next watershed moment we're anticipating in multi-messenger astronomy?
The source that we're all waiting for is a galactic supernova. We see light from supernovae, which are the explosions of stars, all the time. In one case, famously back in 1987, we also detected neutrinos at the same time as the light from a supernova in a nearby satellite galaxy, the Large Magellanic Cloud. If a supernova happens close enough, we expect to also hear gravitational waves. And maybe we'll even detect cosmic rays as well. For this to be observed, though, we need to get very lucky. Supernovae only happen once every century or so in our Milky Way Galaxy. And we need one to be in our neighborhood, not somewhere on the other side of the Galaxy. So we could be waiting quite a while. I wouldn't give this project to a student; they would be unlikely to ever graduate!
Gravitational wave detections were the last piece in the puzzle—at least based on physics as we now know it—for multi-messenger astronomy and are obviously close to your heart, given your work on LIGO. Why was the discovery of gravitational waves in particular such a key event?
I think the discovery of gravitational waves is one of the most beautiful stories in physics. Gravitational waves and black holes were both predicted by Albert Einstein's theory of general relativity a century ago. Both predictions were radical and revolutionary, and contentious at the time. It took 100 years for technology to get to the point where we could build LIGO. These are the most sensitive instruments ever built, and since turning them on in 2015 we've been making all sorts of incredible discoveries, including almost 100 collisions of black holes. The multi-messenger detection of GW170817, however, is in my mind by far the highlight. This will go down as one of the most beautiful and important discoveries in all of physics.