The Lonely Fruit Fly
by Lindsay Borthwick
Fruit flies can help us understand the impact of social isolation, and other highlights from the Kavli Institutes in Neuroscience
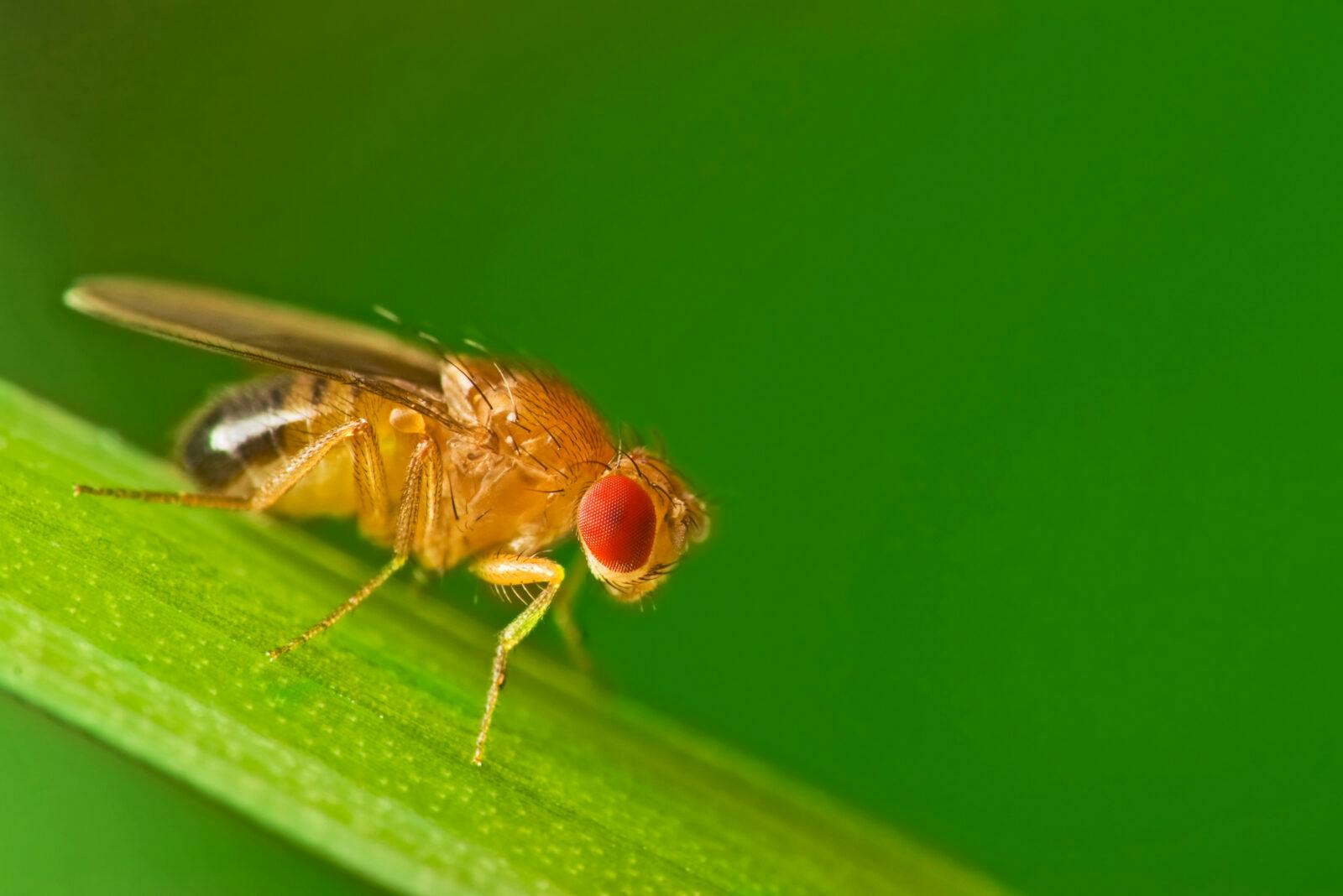
The Author
From New York to Norway, Kavli Institute researchers are making surprising new discoveries about the brain. At Rockefeller University, just seven days of social isolation led to dramatic changes in behavior and gene expression in fruit flies that might help us understand the impact of the pandemic on human health. At the Norwegian University of Science and Technology, a new artificial intelligence tool was able to decode behaviors in rats and humans from raw data, helping researchers more easily interpret brain activity.
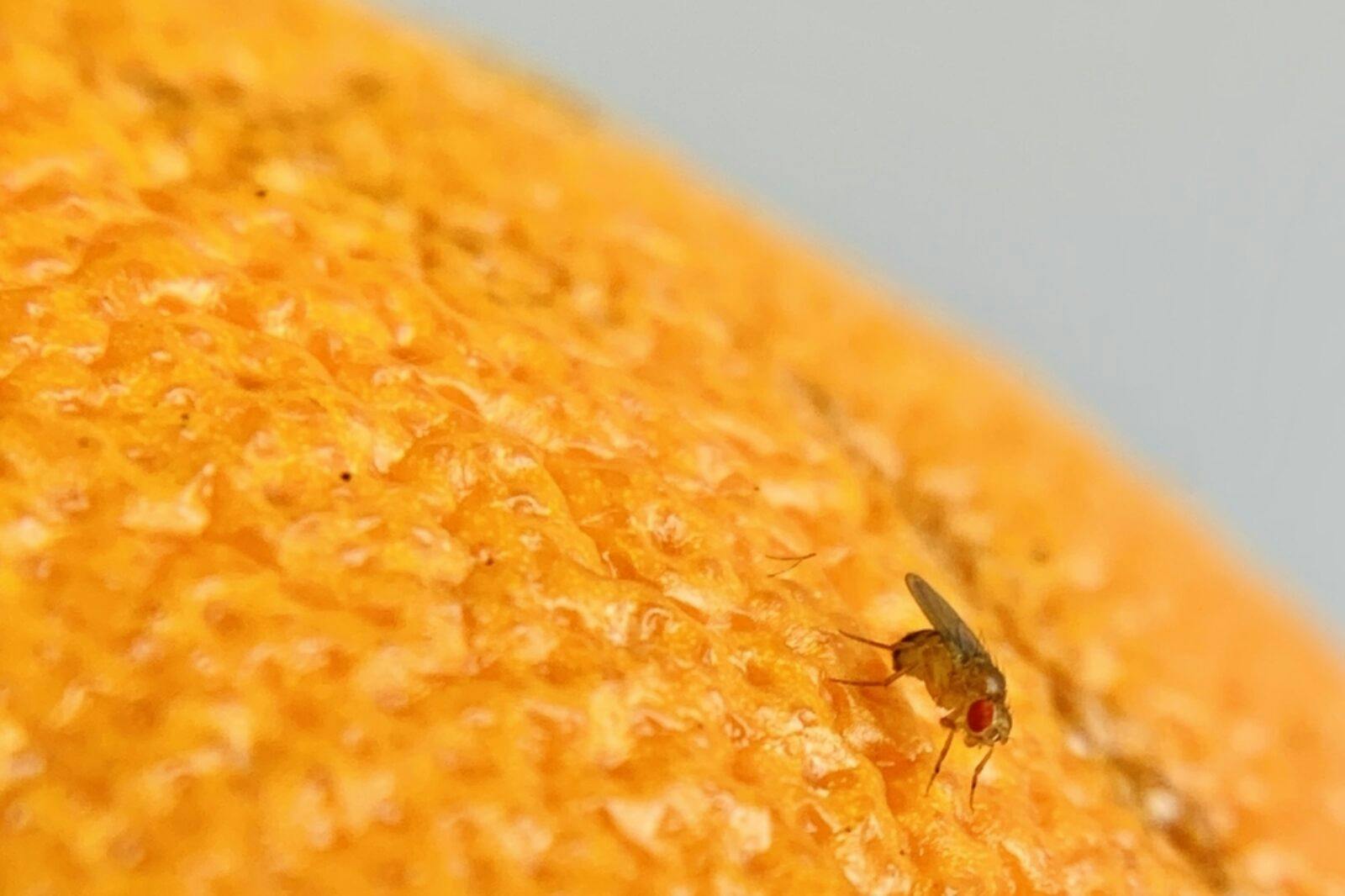
Lonesome Flies
How does social isolation affect the brain? That question that has taken on greater salience during the pandemic, which has torn our social fabric. Now, Michael Young and his team at The Rockefeller University have an answer—at least in fruit flies. In a new study published in Nature, they showed that quarantined flies, which were separated from others for seven days, ate much more and slept much less than flies with a normal social life. Those behavioral changes are strikingly similar to what we see in humans, suggested the flies are a good model for studying the biological impact of loneliness. When the team looked for genetic and physiological changes in the lonely flies, they found gene expression patterns that mimicked those of starvation and a specific type of brain cell associated with the behavioral changes. In a Rockefeller article about the study, Young said: ‘“Clinically-oriented studies suggest that a large number of adults in the United States experienced significant weight gains and loss of sleep throughout the past year of isolation precautions due to COVID-19…. It may well be that our little flies are mimicking the behaviors of humans living under pandemic conditions for shared biological reasons.” Young is a member of the Kavli Neural Systems Institute and a 2017 Nobel Laureate in Physiology or Medicine for the discovery of circadian rhythms.
A Tool for Studying Autism
A new study recapitulates key features of two rare genetic diseases using brain organoids, which are tiny, three-dimensional clusters of brain cells grown in the laboratory. The finding suggests that the organoids, which are generated from skin samples from people with the syndrome, could be a promising model for studying the diseases and potential therapies. People with deletions and duplications to DNA on chromosome 16 tend to have small or large heads. They may also have an autism diagnosis or autistic traits and intellectual disability. When a team led by UC San Diego School of Medicine researchers Alysson Muotri and Lilia Lakoucheva created brain organoids with 16p11.2 deletions or duplications, they found that the size of the organoids mimicked the brain size changes seen in people. The team also used the organoids to home in on a molecular pathway that may contribute to the changes, specifically RhoA, an enzyme with a range of cellular functions that has been implicated in autism and other neurodevelopment disorders. The researchers intend to build on these results: “Our work opens the possibility to therapeutically manipulate the RhoA pathway,” said Muotri, who is a member of the Kavli Institute for Brain and Mind at UC San Diego. “The same pathway may be also damaged in other individuals with autism spectrum disorder who have macrocephaly or microcephaly. Considering this, we can potentially help millions of patients.”
It’s All in the Dose
A tweet thread highlights a new study from the Salk Institute’s Samuel Pfaff. It looks at the role of a microRNA, miR-218, in amyotrophic lateral sclerosis (ALS), or Lou Gehrig’s disease, a motor neuron disease that causes progressive paralysis. MicroRNAs are short molecules that inhibit gene expression, reducing the amount of protein that gets made. miR-218 controls about 300 different genes and is enriched in motor neurons, suggesting it might be important for motor neuron function. However, in people with ALS, it is found at much lower levels. The new study, led by Neal Amon, a former postdoctoral fellow in Pfaff’s lab, found a controlled way to mimic this drop in miR-218 in mice, allowing him to examine the biological consequences of low miR-218 in motor neurons. He found that as miR-218 levels dropped, not all genes were switched off equally. miR-218 affected “different sets of target genes depending on its cellular ‘dose,’” he tweeted. The discovery has implications for understanding other diseases involving changes in gene expression level. “We think that these processes may also take place in other diseases related to genes and aging, including cancer,” said Pfaff, who holds the Benjamin H. Lewis Chair at Salk. “Having a new way to create animal models of how genetic disease begins and how it progresses will allow us to get at the underlying mechanisms and a deeper understanding of these complex activities.” Pfaff is a member of the Kavli Institute for Brain and Mind.
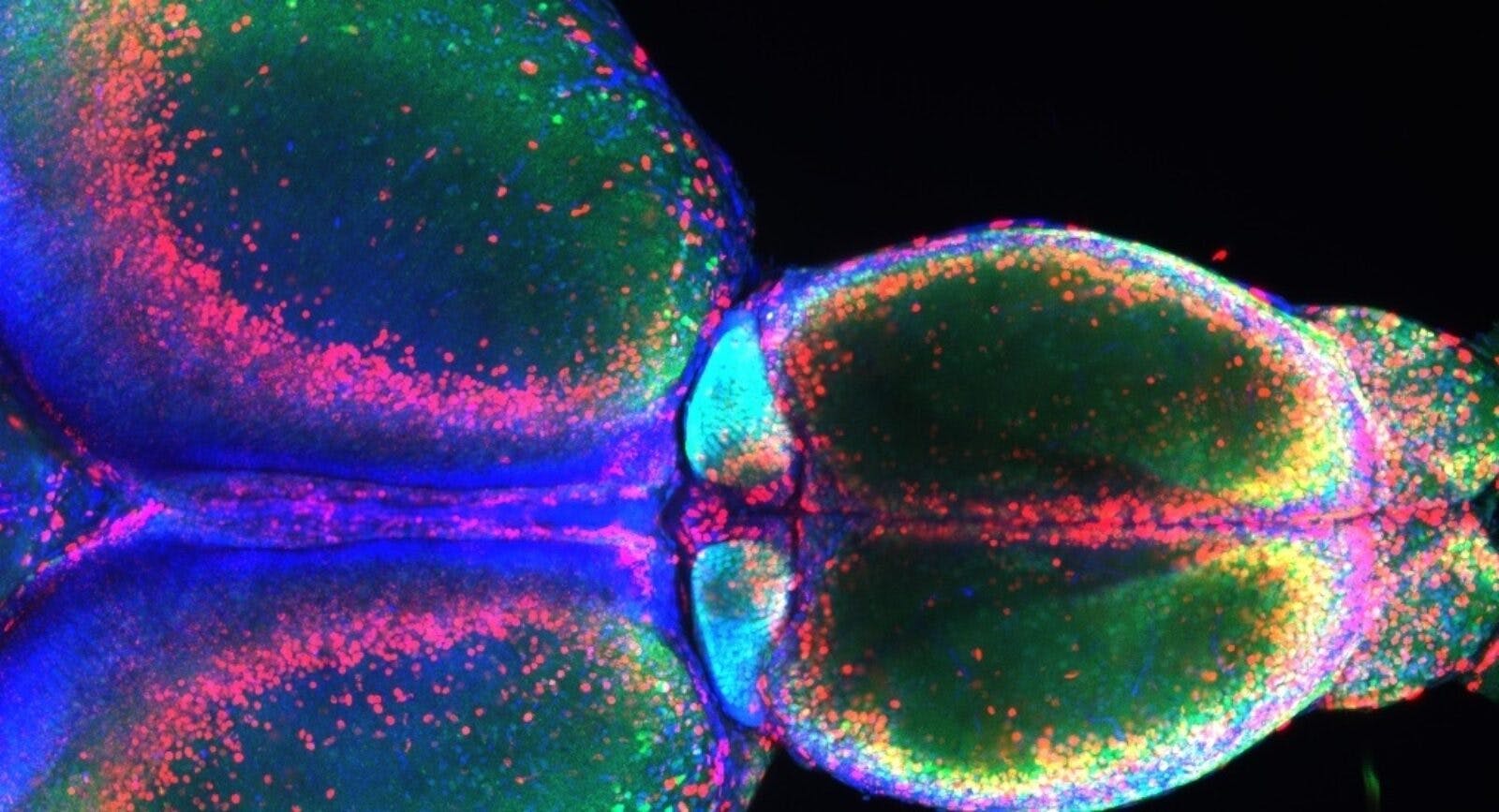
Holy Habenula!
New research from the Kavli Institute for Systems Neuroscience explores the habenula, an area of the midbrain that is conserved across vertebrates, from zebrafish to humans. The habenula is gaining attention because it has been found to play a role in psychiatric diseases, including depression and addiction. Recent studies have found that neurons in the habenula are active during changes in brain state, such as when it goes from being at rest to alert to a potential threat in the environment, but its function is still unclear. Working with zebrafish, KISN’s Emre Yaksi and his team found that the habenula acts as an information hub, integrating sensory signals like odors with information from the limbic system, which guides behavioral and emotional responses. “We argue that the habenula helps the brain to stop certain actions and communications across brain regions, in order to shift it to another mode that is better suited to the situation that the scent warns of,” Yaksi said in an article about the research. “The brain’s different modes involve different dynamic networks that best enable the animal to solve the challenges that it is about to experience.”
Reading Neural Code
Markus Frey, a researcher at the Kavli Institute for Systems Neuroscience, recently showed that a deep learning algorithm called DeepInsight can decode different behaviors from recordings of brain activity. For example, it could predict the position, head direction, and running speed of rats and the hand position of humans just by analyzing brain activity data sets. That power can help researchers automate the analysis of neural recordings, saving time and unlocking new insights into the brain and behavior. Frey is a PhD candidate in the labs of Carswell Barry at University College London and KISN’s Christian Doeller, who is also Managing Director of the Max Planck Institute for Human Cognitive and Brain Sciences.
Neuroscientists have been able to record larger and larger datasets from the brain but understanding the information contained in that data – reading the neural code – is still a hard problem. In most cases we don’t know what messages are being transmitted. “We wanted to develop an automatic method to analyse raw neural data of many different types, circumventing the need to manually decipher them.
Markus Frey