The Mysterious Motion of Water in Plants
by Alan S. Brown
In a time of drought, Abraham Duncan Stroock is looking for new ways to understand how plants use water
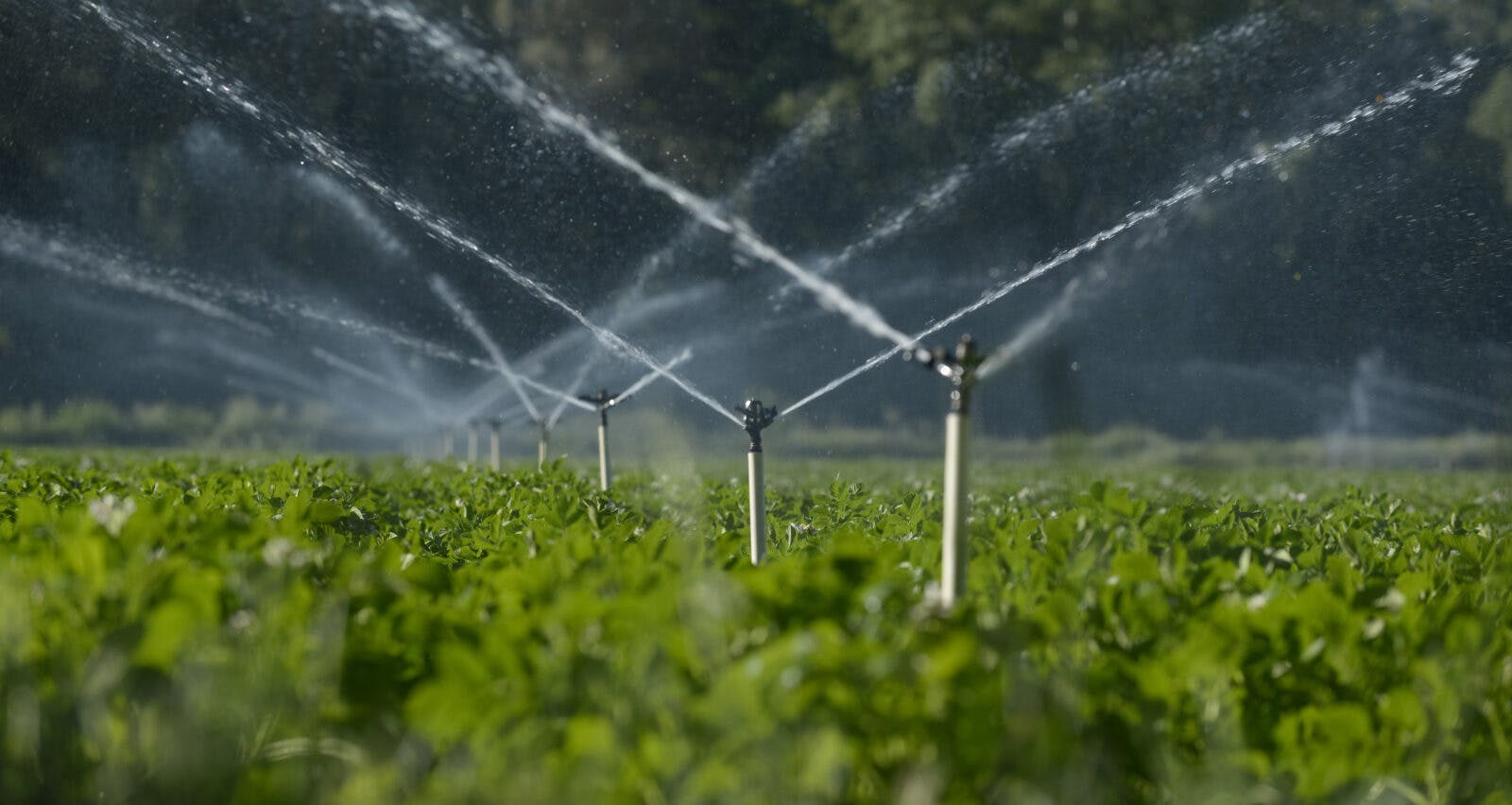
The Author
The Researcher
While fires grab the headlines, droughts pose big problems for agriculture. Growing crops and raising animals accounts for 70 percent of all human freshwater use, explains Abraham Duncan Stroock, a chemical and biomolecular engineer and member of the Kavli Institute at Cornell for Nanoscale Science.
"Farmers face the most catastrophic consequences," he said. "In California, for example, they're tearing out orchards and vineyards. The state is an irrigated desert. If they can't irrigate it, it becomes a desert again."
Fortunately, science can help. Researchers have long used selective breeding, propagating plants that have the best desired traits, to improve plants' ability to survive with less water. Genetic engineers hope to take that one step further, mixing and matching genes to take advantage of the best drought adaptations to create plants that thrive with less water.
Yet, geneticists face a problem, Strook said. They are often unsure what specific traits and molecular mechanisms to target because we know so little about how plants manage water.
"Plants are a black box," Stroock said. "Partly, it's because they are literally an enclosed system in a woody shell. But even the cellular biology of the leaves is extremely difficult to access. We simply do not have the plant equivalent of a hypodermic needle to take a sample."
Stroock wants to create that hypodermic needle. His laboratory is developing tools to look in plants and see exactly where and when water moves through them. With them, plants are finally give up secrets they have kept hidden since the humans first began to cultivate them 12,000 years ago.
Under tension
Stroock started investigating plants as a doctoral candidate under George Whitesides, a pioneer in the field of microfluidics, the movement of small amounts of fluid through tiny vessels. Plants caught Stroock's attention because they did exactly that – but not the way humans did.
Plants release water vapor into the atmosphere, which creates a negative pressure in the xylem, the layer of small vessels that brings water up from the root. If a plant has enough water, it opens its stoma, the pores under its leaves, to pull in carbon dioxide, which it photosynthesizes into sugar to fuel growth.
Stroock was struck by the use of negative pressure, or tension, on plant vasculature. Unlike humans, who push liquid through pipes with pumps, plants use tension to suck up water the way we would with a straw.
The narrow diameter of the microvasculature, however, differs greatly from a straw. It creates enough negative pressure that the water inside those tubes wants to boil away, Stroock said. It would, too, if the microvasculature did not contain it.
"Manipulating water under tension is exotic," Stroock said. "It's not something engineers do, so my research proposal was to build a synthetic system that would allow us to study this as a physics problem."
Eventually, he created a device that models plant microvasculature. Stroock used it to learn how to manipulate liquids with tension. Then he met Alan Lakso, then a professor of horticulture, and realized that his device could be used to measure water in plants.
The tensionometer
Lakso and his fellow researchers were studying the physiology of stress, which is often caused by lack of water. Yet they struggled to trace the movement of water in plants.
This is because plants are incredibly difficult to penetrate. Stroock may joke about plant hypodermic needles, but if he tried to use an actual needle on the fragile filaments carrying water within a plant, he would rip them, and the liquid would boil away.
Imaging doesn't work well, either. Many crops, like grape vines and fruit trees, are encased in a hard wood shell. Even leaves, which seem translucent when held up to the sun, are hard to penetrate. They are loaded with chlorophyll and pigments that scatter light and filled with regions of air and condensed cells that create cloudy contrasts.
To learn what is going inside, scientists sometimes turn to unusual methods.
"Today, in the 21st century," Stroock said, "the best, most reliable way to access the sap carried down from the leaves is to ask an aphid to stick its stylet into the plant. Then you knock it off and collect the little droplets of sap that come out of its body."
Lakso had tools that could accurately quantify water levels in trees and vines, but they were cumbersome and, like a snapshot, only captured the state of the plant frozen in time. Researchers wanted to see in real time how one state morphed into another.
When Lakso and Stroock began talking, they realized Stroock's microfluidic system might provide a solution. Stroock's device, after all, used the same tension-driven principles as the plant itself. If they could interface it with a tree or vine, it would not introduce large pressure changes that would disrupt the plant.
To make this work, Stroock spent years patiently figuring out how to fuse his microfluidic device and its control and measurement electronics with plant tissue.
The device he finally developed looks like a pencil. At its heart is a microfluidic system attached to a sensor on a chip that holds water under tension the way plants do. The sensor is made of a piezoelectric material that generates an electrical current when pressure changes cause it to bend very slightly.
"It's a very simple chip that we can embed in plant tissue without disrupting it significantly," Stroock said. "It's a successful way to watch stress in a plant, minute by minute, and monitor how it changes as plants recover from a hot day, a rainstorm, or something a farmer did. It lets us study the dynamics of the full organism, from kilometers of roots underground to all those square meters of leaves blowing in wind."
AquaDust
In 2014, Lakso and Stroock helped found a company, FloraPulse, based in Davis, Calif., to commercialize the tensiometer for research and agricultural use. They are currently working with nearby almond and grape growers.
Meanwhile, Stroock is also working on a second innovation, AquaDust, that gives researchers an unprecedented way to see how water moves through leaves.
"AquaDust grew out of a desire to zoom in on the most critical part of a plant, where water, light, and carbon come together to drive productivity," Stroock said. "This is where all food in world comes from."
For this project, Stroock teamed up with Mike Gore, a geneticist and plant breeder at Cornell. Gore and other geneticists focus on defining traits they want in future plants, such as drought-resistance, then developing ways to bend and blend plant genes to create those properties.
The information AquaDust provides could provide valuable insights into the mechanisms. "If you can measure something new, you can potentially discover new traits that geneticists and breeders can bring forward to be productive," Stroock said.
Stroock and Gore are focusing on the plant's control system, how it decides how wide to open its stoma. This is always a tradeoff. On one hand, opening wide enables the plant to capture more CO2 to make sugar for energy and growth. On the other hand, opening the stoma could dry out the plant, since it must release hundreds of water molecules for each molecule of CO2 that it absorbs.
"AquaDust lets us zoom in on the leaf with a technology that gives us local access to the critical tissue within the leaves," he said. Unlike the tensiometer, which was designed to interrogate woody trees and vines, AquaDust works with any leaf.
The material itself consists of a fluorescent dye in a hydrogel, a polymer that swells when it contacts water (the way a baby's diaper does). Once squeezed into a leaf through its stoma, the dye expands or contracts, depending on the amount of water present.
Changes in hydrogel volume change the distance between adjacent dyes, which transfer more energy between one another as they get closer. The addition or subtraction of energy changes the color at which they fluoresce in a very predictable way, enabling Stroock's team to create a color-coded map of water availability throughout the leaf.
"Once we get them inside the plant tissue, we can simply shine light and collect the spectrum with a simple field spectrometer," Stroock said. "Then we watch how the availability of water inside the tissue changes."
In essence, AquaDust turns the entire plant into a sensor. Now, Stroock and Gore are working on ways to make it easier to use. They want to collaborate with an industrial company with experience formulating sprays that plants will absorb. If that works, they could then spray AquaDust onto crops for research purposes or to warn of impending water issues. Ultimately, one drone might spray a field while another drone, armed with a spectrometer or hyperspectral camera, takes measurements.
The result, he says, would provide researchers with unprecedented insights into the ways plants use water and provide clues about the genetic mechanisms that make them possible.
In a future of ongoing droughts, engineering plants to make the most out of the water available could be a life-saver for humanity.