The Network Effect
by Lindsay Borthwick
Research Highlights from Kavli Neuroscience Institutes
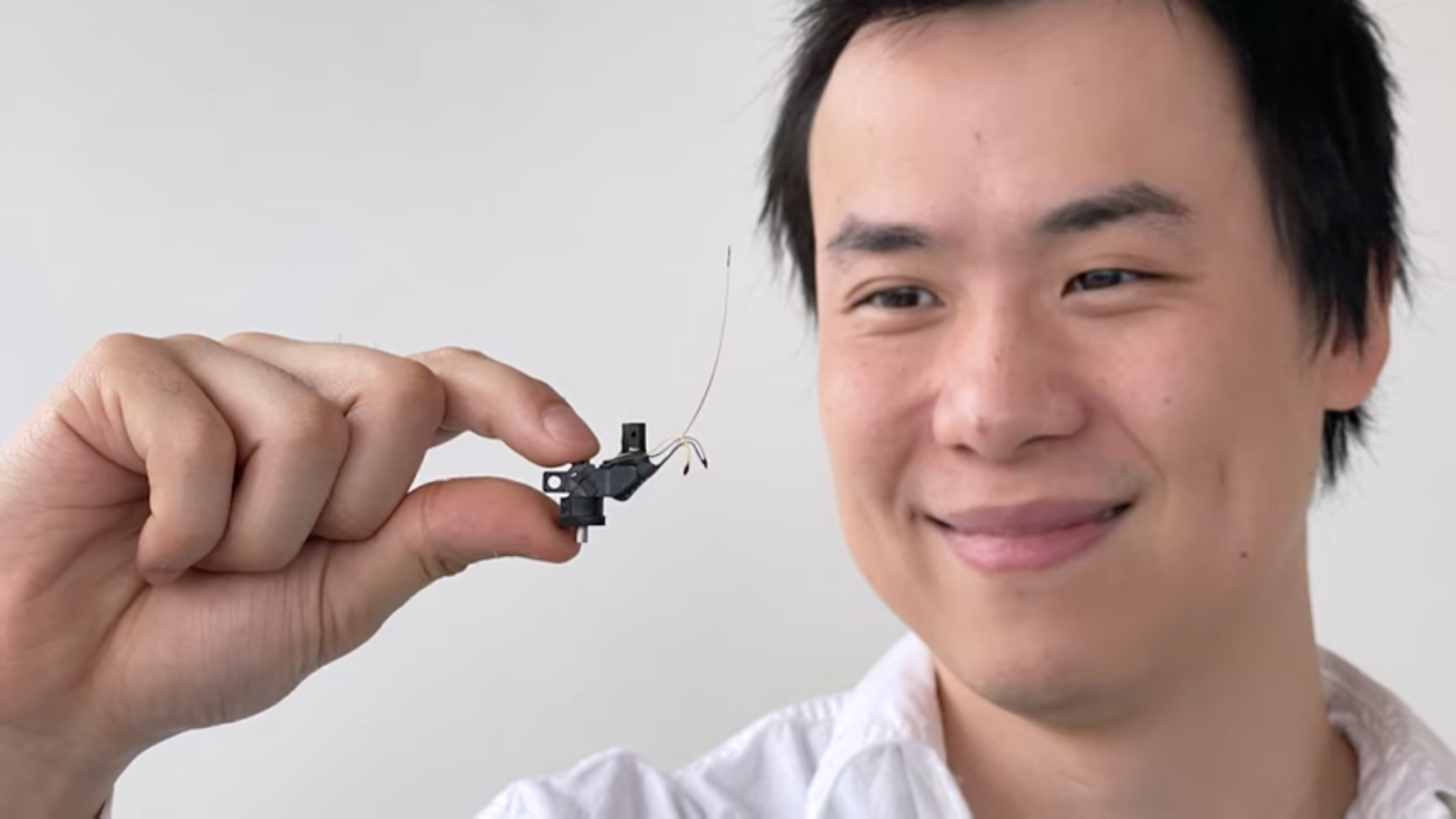
The Author
Networks are at the center of brain research. On the biological level, there are networks of genes, proteins, nerve cells, the connections between those cells locally and at longer ranges, and also the networks of signals they produce. In fact, some have even argued that the field of neuroscience is moving toward “network neuroscience,” where powerful new tools are helping researchers uncover different kinds of networks and study how influence brain function. At the community level, there are also networks of researchers working together in small groups or large international consortia to move brain science forward. In this roundup, we’re taking a closer look at a handful of different neuroscience networks and the impact they are having on the field.
The Mini2P Community Network
There are astronomical observatories scattered around the world that reveal the cosmos. Now, there is also cortical observatories, made possible by a tiny, portable, lightweight microscope that provides a window into the brain in action. The new device, named the Mini 2P, was designed by researchers at the Kavli Institute for Systems Neuroscience at the Norwegian University of Science and Technology. It can be fixed to a mouse’s head and used to record the animal’s brain activity as it thinks, plans, feels and remembers. The device measures light emitted from brain cells that have been engineered to fluoresce when active. The Mini2P is an example of a new generation of microscopes that provide a view of constellations of brain cells and could yield insights into how the brain gives rise to complex behaviors. A paper describing Mini2P—which is an open-source tool built by and for the brain research community—was recently published in the journal Cell. “We believe the Mini2P is a game changer, and we want to share it with neuroscientists and labs across the world,” said May-Britt Moser, Ph.D., Co-Scientific Director of the Institute.
Cellular Networks Shaped by Evolution
How the human brain evolved is still a mystery, but researchers pinpoint some of the developmental changes in the primate brain that could explain it. A new study led by neuroscientist Alex Pollen, Ph.D., catalogs the diversity of inhibitory neurons early in the developing primate brain. Inhibitory neurons (INs) dampen the flow of information in the brain and are a small but crucial class of neurons in the cerebral cortex. Researchers studying brain evolution are especially interested in INs because, compared with other mammals, the cells are abundant in the human cortex. When Pollen and his team examined what types of INs are present early in development, they identified just 16 classes from which all classes of adult INs eventually arise. They also found that the suite of INs in the developing brain is very similar to those found in the developing mouse brain. This suggest the major evolutionary differences between the mouse and primate brain arise later in development, as the brains mature. Though the cell types were well-conserved, the researchers discovered a new type of striatal IN, which had recently been identified for the first time in the adult primate brain. “Where rodents seem to have this one striatal interneuron type that has receptors, now primates have two, and they have seemingly different roles in terms of how they might function in a circuit,” said first author Matthew Schmitz, a Ph.D. candidate in Pollen’s lab during a recent conversation with The Kavli Foundation. Now that they have a more complete parts list for the developing primate brain, the researchers are going to continue to study how INs influence its unique function. Pollen is an assistant professor at the University of California, San Francisco, and a member of the Kavli Institute for Fundamental Neuroscience.
The Biology of Social Networks
Social rank is important. Anyone who has ever seen chickens in action know that they fiercely defend their place in the pecking order. Well mammals do, too. Neuroscientist Kay Tye, Ph.D., set out to understand how the brain encodes social rank and how rank influences everyday behaviors, such as who eats first in a group. In a series of experiments in mice, Tye and her team pinpointed the cells in the medial prefrontal cortex (mPFC) involved in social dominance. Then, by measuring and decoding the activity of those cells, they were able to predict which animal would outcompete the others for a food reward, even before the animals had moved a muscle. Surprisingly, they found that the winner wasn’t always the animal with the highest social rank; rather, it was the one with the winning mindset. In an interview, Tye said that by revealing how a group of cells gives rise to a mindset, the study helps bridge psychological science and neuroscience, or mind and brain. “Most social species organize themselves into hierarchies that guide each individual’s behavior,” said Tye, a professor at the Salk Institute, where she is affiliated with the Kavli Institute for Brain and Mind. “Understanding how the brain mediates this may help us understand the interplay between social rank, isolation, and psychiatric diseases, such as depression, anxiety, or even substance abuse.” The research was published in March in the journal Nature.
Networks of Attention
In a research lab, there is a slew of ways to measure attention—a process essential to our ability to perceive and understand the world around us. There isn’t a single measure because there are different types of attention, such as the ability to focus on a task for a period of time and to temporarily hold upcoming tasks in mind. Researchers affiliated with Yale University’s Kavli Institute for Neuroscience, wondered whether they could analyze brain scans to determine what various attentional tasks have in common, and whether they could use that information to quantify a person’s general ability to pay attention. The answer turns out to be yes. The researchers collected fMRI data from about 90 people at rest and as they performed various attention tasks. From those data, they created a model that could predict a person’s performance on attention tasks. The model hinged on finding a way to analyze patterns of the brain networks from the scans. “The brain is all interconnected, and is always running like a beating heart,” said Marvin Chun, Ph.D., a professor of psychology and neuroscience, co-corresponding author on a new study published in the journal Nature Human Behavior. “What we can do is take all those complex patterns and analyze the data to create a fingerprint of the brain’s ability to pay attention.”
The Brain-Body Connection
We often thinking of our senses as a conduit to the outside world. But the brain also needs to be able to sense our inner world—the ever changing state of our body, including our internal organs. Rui Chang, Ph.D., is focused on understanding the neural interplay between the brain and body and how it is altered in various diseases. In a new study published in the journal Nature, Chang and his team wondered: How do neurons in the vagus nerve, which connects the brain to all the major organs, send life-sustaining signals to the brain from all over the body? And how does the brain discriminate those signals? They found that vagal sensory neurons encode three distinct features into a signal: the organ, the tissue layer and the stimulus. For example, a signal originating in the stomach carries information about the organ itself, plus whether it is originating in the surface connective tissue, muscular layer or mucosal lining; it also encodes the stimulus, such as stretching or nutrient influx from ingested food. “This is the first time we actually know how different body signals are being represented through the vagal interoception system to the brain in a very precise and accurate manner,” Chang told Yale News. “We know that the brain can very precisely discriminate signals, but what is the biological reason for that discrimination?” Chang, who is a member of the Kavli Institute for Neuroscience at Yale, hopes to take advantage of the new discoveries to design tools to target individual signaling pathways within the vagus nerve.