The Neuroscience of Motivation
by Lindsay Borthwick
Research at Kavli Neuroscience Institutes spans athletic performance to decoding speech
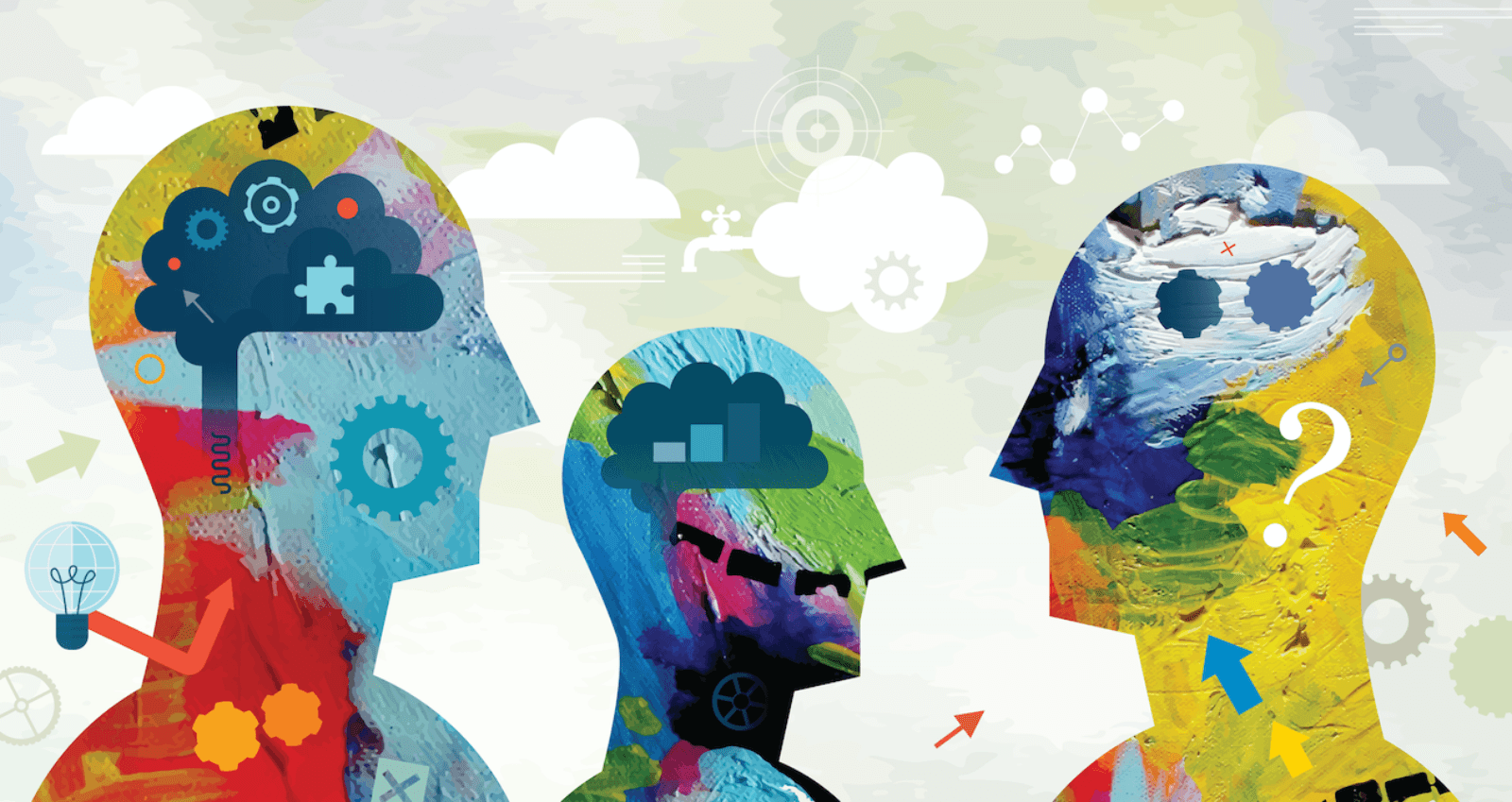
The Author
The neuroscience of motivation bookends this roundup of research highlights from the Kavli Neuroscience Institutes. It begins with a Q&A with Vikram Chib, a researcher at Johns Hopkins’ Kavli Institute for Neuroscience Discovery, who discusses whether athletic performance will suffer at the Olympic Games without fans in the stands. The roundup ends with a surprising finding about dopamine, a feel-good neurotransmitter implicated in various cognitive processes including motivation. The research, led by David Kleinfeld at the University of California, San Diego, home of the Kavli Institute for Brain and Mind, focuses on spontaneous dopamine impulses that are released in the brain in the absence of a reward. We also cover research that proves speech can be decoded from brain signals, waves that help build the brain, and a fly’s neural switchboard. Are you motivated to read on?
Motivation Maxim
This summer’s Olympic Games in Tokyo are like a living laboratory for Vikram Chib, a professor of biomedical engineering and member of the Kavli Neuroscience Discovery Institute at Johns Hopkins University. Chib studies the neural processes underlying motivation and performance, and how they are connected. This line of research is not only relevant to high-performance sport but also to health because a loss of motivation is associated with psychiatric disorders like depression and schizophrenia. In a recent interview in the lead up to the Olympics, Chib said, “The thing about motivation is, it's like medicine. You want to get the right dose.” Consider fans at a sporting event. Spectators at an Olympic venue provide a social incentive that may boost an athlete’s performance. On the other hand, Chib’s research has shown that too much pressure, say, in the form of a massive crowd, can cause a person to choke. So, how does he think Tokyo 2020’s fan ban will affect the athletes’ performance? “I don't think it will make a big of a difference. Maybe that's not the sexiest answer—but I don't think it's going to make much difference,” said Chib.
A Very Good Day
In an experiment that wowed the world, a team of researchers at the University of California, San Francisco proved it is possible to decipher speech from the brain’s electrical signals. Led by neurosurgeon Edward Chang, who is a member of the Kavli Institute for Fundamental Neuroscience at UCSF, the researchers worked for more than a year and a half with a paralyzed man, nicknamed Pancho, who has lost the ability to speak. Using an electrode implanted in the part of his brain that would control the movement of the vocal tract, they recorded his brain signals as he was thinking words and sentences in response to a prompt, such as “How are you today?” A machine learning algorithm was used to analyze the signals and was able to decode Pancho’s response to that question, captured in the video above. Chang reported the results in a study published in July in the New England Journal of Medicine. In a New York Times article about the research, Pancho said participating in the research has been “a life-changing experience.”
Life Simulator
About a decade ago, neuroscientist Michael Crair described waves of activity traveling through the brains of neonatal mice. The waves originated in the retina—at a time when the mice’s eyelids were still closed and it was deprived of visual cues—and propagated through the brain to other areas. Crair showed that the waves helped establish the circuitry of the visual system and those other areas. Now, he has published a related study that helps to answer the question, how can a newborn mouse make sense of the world the minute it opens its eyes for the first time? In the new study, his research found that the waves of activity mimic those that would be produced as the animal moves forward in the real world. It is as if the waves are helping the unborn animal prep for life after birth. “These brain circuits are self-organized at birth and some of the early teaching is already done,” said Crair, who is a professor of neuroscience and a member of the Kavli Institute for Neuroscience at Yale University, in a news article. “It’s like dreaming about what you are going to see before you even open your eyes.” The team also investigated the waves’ origin, which turns out to be star-like neurons in the retina. Inhibiting these cells prevents the waves from forming as well as the animal’s ability to respond to visual motion after birth, underscoring how important they are to wiring up the brain.
Sensory Switch
A new study
from Vanessa Ruta’s team at The Rockefeller University sheds light on a fundamental question in neuroscience: How does the brain switch the way it reacts to the same stimulus? For example, the sight of a female fruit fly can spark an elaborate courtship ritual in a male fly, or it can go virtually unnoticed. The difference is the fly’s state of arousal. To explore this switch, Ruta and her team recorded brain activity in male fruit flies as they observed virtual ‘female’ flies projected onto a screen. Ruta’s team found that a collection of brain cells called P1 neurons act as a kind of neural switchboard in the males. When the fly is aroused by the sight of a flying ‘female’ and its P1 neurons are active, the cells route sensory information from the visual system to the motor system—and the courtship can begin. When the P1 cells are inactive, the fly's visual-motor circuit stays dormant, effectively rendering the males blind to the nearby female. “This design ensures that the fruit fly doesn’t engage in courtship all the time, but only under the right conditions,” Ruta said in news article. “In this way, a behavior like courtship, which is genetically programmed, can be at once robust and flexible.” Ruta is head of the Laboratory of Neurophysiology and Behavior and a member of the Kavli Neural Systems Institute at Rockefeller.
Mysterious Impulses
Dopamine is like the Swiss Army knife of neurotransmitters: It is involved in movement as well as cognitive processes like motivation, reward and reinforcement. The brain is flooded with dopamine in response to an unexpected reward, making us feel good, and motivating us to seek that reward again. But it turns out that brain cells in mice receive spontaneous pulses of dopamine in the absence of a reward—an intriguing finding that David Kleinfeld, his graduate student Conrad Foo and their collaborators explored in a series of experiments. They sought to understand whether the animals were aware of these random dopamine impulses and also whether they could control them, in much the same way that dopamine levels are elevated in animals that anticipate a big reward. Surprisingly, they found that the mice are aware of the pulses and can wilfully manipulate them. The team suspects that these bursts of dopamine help mice with behavior planning such as foraging or finding a mate—behaviors that are essential to survival. Kleinfeld is a professor of physics and of neurobiology at the University of California, San Francisco, where he is also affiliated with the Kavli Institute of Brain and Mind.