The Not-So-Secret Lives of Cells
by Lindsay Borthwick
Newfound ways of peering into cells help researchers unlock the mysteries of the brain
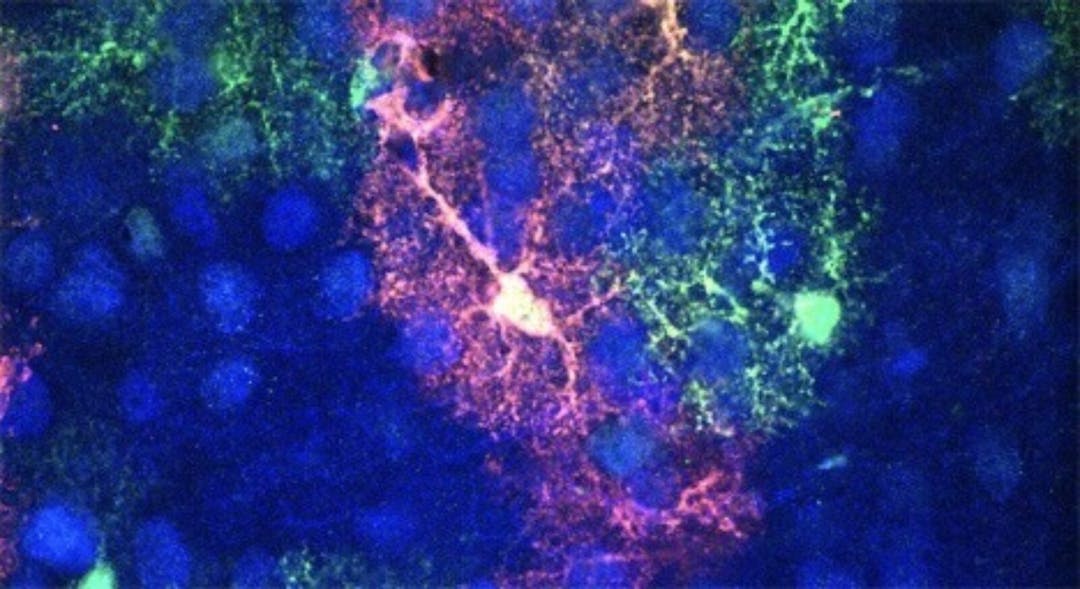
The Author
Newfound ways of peering into cells are helping Kavli-affiliated researchers unlock the mysteries of the brain. Take, for example, a remarkable new super-resolution microscope that can visualize the inner life of cells in live mice. Researchers at the Kavli Institute at Yale are using it to peer at dendritic spines, highly dynamic structures on nerve cells that receive and modulate signals from nearby cells. In different study, Yale researchers traced the developmental origins of human cells back to the very first cell divisions following fertilization by looking at the subtle differences in their genetic sequences. And, a team from the University of California, San Francisco discovered that non-neuronal cells in the brain called astrocytes may hold the secret to sleep. The cells have a far-reaching effect on the activity of neurons that helps tip them toward the Zzzzs.
Sleep-inducing cells
Astrocytes, star-shaped non-neuronal cells in the brain, have a powerful influence on sleep, according to new research from Kira Poskanzer, PhD, and her team at the University of California, San Francisco. Yet, until now, there has been scant research on the role of these brain cells in sleep. “What have people been missing because they’re ignoring this group of cells?” Poskanzer asked in a news release. “The questions that haven’t been answered thus far in sleep neurobiology – maybe they haven’t been answered because we haven’t been looking in the right places.” When her research team activated astrocytes in the brains of mice, they measured more slow-wave activity—brain waves that smoothly oscillate from high peaks to low troughs—evidence that the astrocytes help tip the brain toward the state of sleep. They could also influence the depth and duration of the animals’ sleep by activating certain receptors on the surface of the cells, a finding that has implications for the treatment of sleep disorders. Poskanzer is a member of the Kavli Institute for Fundamental Neuroscience.
Cellular roots
When a human egg is fertilized, it divides into two cells called blastomeres. Those two cells keep on dividing, eventually forming a human being composed of trillions of cells, hundreds of which have distinct specialized functions. As those cells divide, they accumulate somatic (non-inherited) mutations that serve as markers that can be used to trace the lineage of a cell—its family history. Working backward, researchers at Yale the Mayo Clinic recently reconstructed the histories of cells from two adults to understand how human development plays out, even at its earliest stages. “It’s like Ancestry.com for our bodies,’’ said Flora Vaccarino, MD, a professor of neuroscience at Yale University and co-senior author of a new study published in the journal Science. The research could allow scientists to trace developmental disorders, such as autism, back to their cellular roots, and to understand why the signs and symptoms of some diseases present asymmetrically. In a striking example of asymmetry in cell division, the researchers found that up to 90 percent of blood cells originate from one of the first two blastomeres. Vaccarino is a member of the Kavli Institute for Neuroscience at Yale.
Super-resolution imaging
A new microscope can image dendritic spines deep inside the brain of a living mouse. Dendritic spines are the small neuronal protrusions that form the basis for connection, or synapses, with other nerve cells, influencing how information is processed and stored in the brain. Built by physicist Joerg Bewesdrof, PhD, and his team at Yale University, the new super-resolution microscope builds on Nobel Prize-winning research that showed that scientists could overcome the laws of physics to visualize biological structures at the nanoscale. Using the new device, known as a 3D-2PE-STED microscope, the researchers were able to zoom in on a dendrite, see the 3D structure of individual spines and track how their structure changed over time. Bewersdorf is a member of the Kavli Institute for Neuroscience at Yale.
Lessening loneliness
Loneliness doesn’t just have psychological consequences. Evidence is mounting that it can also undermine health and longevity. That is why researchers like University of California, San Diego’s Jyoti Mishra, PhD, are studying the relationship between the brain, the mind and urgent societal issues like loneliness and trying to find ways to intervene. In a new study, Mishra and her colleagues, including Dilip V. Jeste, MD, showed that specific brain regions respond to emotional stimuli related to loneliness and wisdom in opposing ways. For example, lonelier people tended to react to negative stimuli like an angry face more strongly than others—an emotional response that was accompanied by greater brain activity in a region known as the temporal-parietal junction. “These findings… give us an objective neurobiological handle on how lonelier or wiser people process information,” said Mishra. “Having biological markers that we can measure in the brain can help us develop effective treatments. Perhaps we can help answer the question, ‘Can you make a person wiser or less lonely?’ The answer could help mitigate the risk of loneliness.” Both Mishra and Jeste are affiliated with the Kavli Institute for Brain and Mind.
Touch trap
The touch-sensitive Venus flytrap quickly snaps shut to ensnare its prey. But exactly how it senses that prey and responds to touch isn’t well understood. Now, researchers at the Salk Institute and Scripps Research have discovered a new protein, FLYCATCHER1, that triggers the plants’ response. It is the second most abundant protein in the hairs that line plants' leaves. When the researchers expressed FLYCATCHER1 in mammalian cells, they found that the cells generated electrical currents in response to touch, persuasive evidence that the protein is sensitive to mechanical stimuli. They also genetically modified the plants so FLYCATCHER1 was missing. As a result, the mutant flytraps could no longer sense touch. The results have implications for understanding how plants detect and respond to mechanical stimuli and might even have applications in medical technologies that stimulate neurons and other human cells. The senior co-authors on the study are plant biologist and geneticist Joanne Chory, PhD, and Ardem Patapoutian, PhD, co-recipient of the 2020 Kavli Prize in Neuroscience for the “transformative discovery of receptors for temperature and pressure.”