A Roundtable Discussion with the Kavli Institute for the Physics and Mathematics of the Universe
At the newest Kavli Institute, solving the biggest mysteries of the cosmos is a "multilingual" enterprise where the research in mathematics, physics and astronomy combine to create a more complete understanding of the universe.
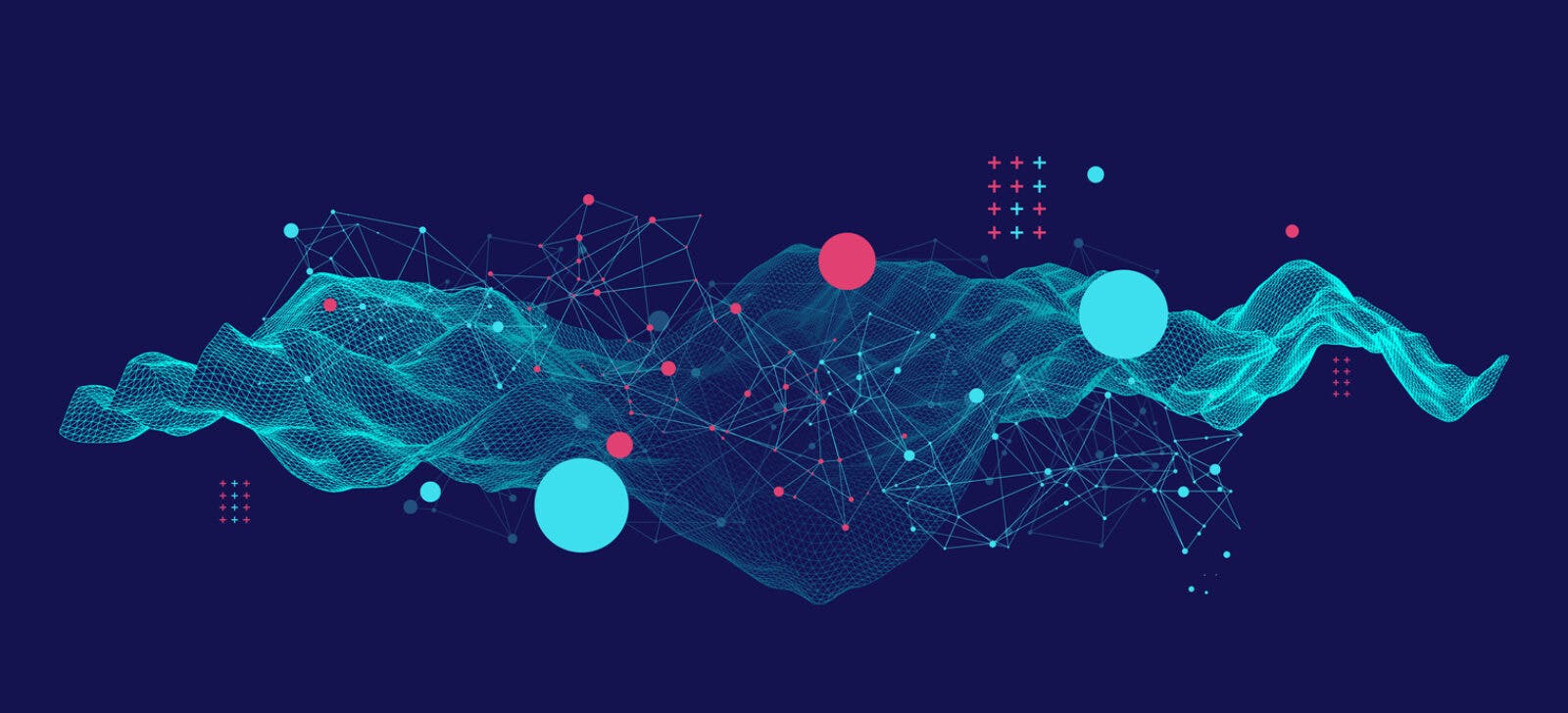
The Researchers
TACKLING THE BIGGEST QUESTIONS in cosmology requires a collective intellect. Scientists from fields as diverse as string theory and mathematics to particle physics and astronomy must work together for humanity to gain a fuller understanding of the cosmos in which we live.
The Kavli Institute for the Physics and Mathematics of the Universe at the University of Tokyo (Kavli IPMU) is designed to bring all these researchers under one roof. The Kavli IPMU hosts about 200 researchers from a diversity of fields to tackle many of the most challenging problems in cosmology, including the mysteries of dark matter and dark energy, which together make up nearly 96 percent of the universe yet are completely unknown. These researchers are focused on 15 different areas, such as alternative gravity theories, pure mathematics, particle astrophysics, dark matter experiments, and observational cosmology. About half of its researchers come from outside Japan – reflecting the importance of drawing together excellent scientists from around the world..
Established in 2007 as one of five premier science research centers by the Japanese government, the Institute became a Kavli Institute this year in recognition of an endowment from The Kavli Foundation. In late January, the Institute’s director, Hitoshi Murayama, joined with deputy directors Hiroaki Aihara and Yoichiro Suzuki to discuss the latest research at the Kavli IPMU, what’s in store over the coming months, and the collaborative and international atmosphere that shapes daily life at the Institute.
- Hitoshi Murayama – Director of the Kavli IPMU, Murayama is also the MacAdams Professor of Physics at the University of California, Berkeley and a senior staff scientist at Lawrence Berkeley National Laboratory. A particle physicist and theorist, he works on a wide range of subjects related to physics and cosmology, developing strategies for new particle experiments; studying neutrinos, fundamental particles which are among the least understood in the universe; developing new dark matter models; and other endeavors.
- Hiroaki Aihara – Deputy Director of the Kavli IPMU, Aihara is also a Professor in the Department of Physics at The University of Tokyo and former researcher at the Lawrence Berkeley National Laboratory. A particle physics experimentalist, Aihara contributed significantly to the discovery of the elementary particle, top quark, in 1995. His current research includes the study of fundamental particles of nature and working on the large wide-field survey of galaxies called SuMIRe (Subaru Measurement of Images and Redshifts).
- Yoichiro Suzuki – Deputy Director of the Kavli IPMU, Suzuki is also a Professor of the Institute for Cosmic Ray Research (ICRR) at the University of Tokyo, as well as the director of ICCR’s Kamioka Observatory. A particle physicist, Suzuki proposed XMASS, an underground dark matter detector in Japan. Suzuki is the winner of the 2010 Bruno Pontecorvo Prize, the 2001 Nishina Memorial Prize, and the 1999 Asahi Prize for his work on neutrino physics. He is also a spokesperson for the Super-Kamiokande experiment, an international project studying neutrinos.
Below is an edited transcript of the discussion.
HITOSHI MURAYAMA: I guess the most immediate exciting thing about the upcoming year is actually what Hiroaki can talk about: the HyperSuprimeCam, or HSC. That's the new digital camera on the Subaru telescope at Mauna Kea, Hawaii.
HIROAKI AIHARA: For the upcoming year, we're looking at First Light for the new camera. This new camera will help with our next-generation galaxy surveys to learn about dark energy, which is the unknown force that is accelerating the expansion of the universe and whose discovery led to the Nobel Prize in Physics late last year. It's going to be a wide and deep survey. We plan to cover about 2,000 square degrees of sky, and hopefully we can observe about 200 million galaxies through this survey. Our survey will cover about one-tenth of the sky above the Northern Hemisphere.
MURAYAMA: If you look at the assembly of this camera, it's actually huge. It's about three and a half meters high.
AIHARA: And it weighs three tons and has 900 million pixels.
TKF: Dr. Murayama, will you be involved in work there as well?
MURAYAMA: Yes, the first step is to use the HSC for imaging. We will follow that with spectroscopy later, probably five years from now, and the combination of these efforts we call SuMIRe, which stands for “Subaru Measurement of Images and Redshifts.” The project, combining wide-field imaging and detailed measurements of galaxy redshifts, aims to explore the nature of dark energy and the growth of structure on cosmological scales. I have put together an international collaboration to build the spectrograph with researchers at Caltech, Princeton and Johns Hopkins in the United States; Le Laboratoire d’Astrophysique de Marseille in France; The Institute of Astronomy, Geophysics and Atmospheric Sciences (IAG) and the National Astrophysics Laboratory (LNA) in Brazil; and Academia Sinica Institute of Astronomy and Astrophysics (ASIAA) Taiwan. It's actually gaining a lot of momentum now. We're headed to the review of this project in March so we are working very hard toward that.
TKF: So are you personally involved in the design of that spectrograph?
MURAYAMA: Yes, I am. I am actually not the technical person to do it, but I am leading the team.
TKF: What else are you looking forward to in 2012?
MURAYAMA: Another thing that actually Yoichiro Suzuki should chime in about is the XMASS project. It's an underground experiment looking for dark matter directly.
YOICHIRO SUZUKI: XMASS is designed to look for dark matter by using 100 kilograms of liquid xenon. Construction of the detector has been completed, and we are commissioning it now. We hope to begin gathering data this year.
TKF: IPMU was designed to be a multidisciplinary research campus, where theorists and experimentalists, particle physicists and observational astronomers, are all under one roof. Why is this so important?
MURAYAMA: The answer is very simple: Mathematicians alone, theoretical physicists alone, astronomers alone or experimental physicists alone cannot solve any of the big mysteries of the universe. They have to work together. Let me give you an example at IPMU. As mentioned already, we are building toward First Light for HSC and following up with spectroscopy later on as part of our quest to understand dark energy. It's a huge mystery, as you know. The universe is accelerating, and we have to understand what is behind it. But the galaxy survey by itself won’t tell us. String theorists also have something to say, because this has to do with quantum gravity, and of course astronomers wouldn’t know anything about quantum gravity theory. String theorists are actually working on that, but they don’t know anything about observation. So “forcing” them to talk to each other and think about the same problem together gives us a great advantage over many other institutes around the world.
There are many other examples for why collaborations are essential. The universe is getting bigger at an accelerating rate. But if you go back in the past, the universe was once so small that eventually the whole universe was a single point. That is actually a problem for physical theories because if everything in the entire universe was concentrated at a single point, density was infinite. And infinity is something that physical theories cannot deal with. However, mathematicians have been talking about infinities and singularities all along. That's actually one of the very common themes in geometry. So they have something to say about how to resolve singularities, and that's how the theoretical physicists and the pure mathematicians can actually talk to each other and try to address the problem of how the universe began.
"The answer is very simple: Mathematicians alone, theoretical physicists alone, astronomers alone and experimental physicists alone cannot solve any of the big mysteries of the universe. They have to work together." – Hitoshi Murayama
TKF: What specific projects have particularly benefited from this cross collaboration?
MURAYAMA: One example is collaboration we have between theoretical physicists and mathematicians. As you probably know, string theory is regarded as the candidate for a unified theory of physics in the universe – a theory that would explain how the universe works at both quantum and cosmological scales. String theory is a highly mathematical theory, so when you try to extract some predictions out of string theory you have to delve into pretty high-level mathematics.
Our universe is four dimensional, with three dimensions of space and one dimension of time. But string theorists believe that the universe is actually 10 dimensional, so there are six more spatial dimensions that are curled up in small sizes and hidden from our view. It turns out the shapes, structure and size of this extra six dimensional tiny space give you predictions on the nature of physics in the observed existing four-dimensional universe.
So you have to know something about this six dimensional geometry. Taizan Watari, a theoretical physicist, wanted to grapple with this question and he ended up talking to a mathematician here, Yukinobu Toda, who works on algebraic geometry. Yukinobu helped Taizan analyze some of these six dimensional geometries and he got concrete results out of it. Neither of them could do it individually, but together they could.

Another example is actually what I am doing right now. I'm trained as a theoretical particle physicist, but now I'm actually working with a team of instrument builders so I can address the questions of dark energy. And so I am motivated from the theoretical side to understand this mystery, but on the other hand the observational astronomers would also like to get data to address these questions. At the same time instrumentalists would like to design a very exciting instrument that they can build. And so that's the kind of attack team of instrumentalists, observers, and theorists that is working together toward a single aim.
TKF: All three of you are particle physicists, but your work has also crossed into other fields. Dr. Murayama just described one of his projects; Drs. Aihara and Suzuki, can you each speak a bit more about your collaborations across disciplines? How does your work in one field help inform work in the other?
AIHARA: I'm an experimental particle physicist by training, and dark energy is really a fundamental question for particle physics. Yet, we don't have a way to produce dark energy, and the only way to study dark energy is through astronomical observations. So naturally, because I'm interested in dark energy from a fundamental point of view, I have to collaborate with astronomers. This problem is so hard that we have to mobilize everybody – particle physicists, even mathematicians. Although I am still struggling with how to work with mathematicians (laughs). Still, I may be able to learn something about dark energy from their point of view.
TKF: Dr. Suzuki?
SUZUKI: Dark matter is a major ingredient of the universe, and it must be an elementary particle that has yet to be discovered, so I think collaborations among astrophysicists and theorists are very natural.
I have a general comment about cross collaborations. A cross collaboration at IPMU is a kind of new trial and test. And I do not think we need to specify the outcomes. We have people from different fields – mathematicians, theorists, experimentalists – and at the beginning they may have their own languages. Even though they talk about the same things, they can't communicate with each other at the beginning. But as we put them together, they begin to communicate and understand each other. As a consequence we will naturally have important achievements that are outcomes of IPMU.
"[The] IPMU is a completely new type of institute that will be a model for future scientific institutions in Japan. I hope that IPMU will produce important results that nobody today can imagine." – Yoichiro Suzuki
MURAYAMA: I couldn't agree more. Let me chime in on a few more points about this. One thing we really cared about when we designed this Institute was to put in an infrastructure that encourages this interdisciplinary interaction. So we have offices from the 3rd to the 5th floor. And the traditional thinking of the building would go something like this: let's put mathematicians on the 3rd floor, physicists on the 4th floor, and astronomers always want to be as close to the stars as possible so let's put them on the 5th floor, right? But we insisted with the architectural designer that we wanted to put everybody on the same floor; otherwise they wouldn't bump into each other except in elevators and that wouldn't promote interaction. And the designer made it happen. The way he arranged offices is that if you just keep walking the corridor of the 3rd floor, the floor actually ramps up and you find yourself on the 4th floor. And it ramps up again and you find yourself on the 5th floor. Everybody is really on the same floor, so people actually bump into each other just passing in front of other people's offices.
We also mixed up offices as much as possible, so if in one office there's a mathematician the next office will have an astronomer, and the next one will have a theoretical physicist and so on.
Also, everybody has a duty of coming to teatime at 3 o'clock at the center of this building to mingle. And people share seminars and workshops. So, we have mechanisms to force people to get together and interact with one another, and that really helps people to understand each other's language, which is what Yoichiro was referring to. We’ve made big progress in promoting this cross-cultural, cross-disciplinary research environment.
TKF: The Institute made the news in 2011 with new computer simulations of the appearance of the first stars, and also for new observations of vigorous star formation in one of the most distant and earliest galaxies seen. How do you think these two groups of researchers at IPMU, modelers and observational scientists, help one another to advance their work?
MURAYAMA: The most important thing is for our researchers to give each other inspiration and motivation. The example you mentioned is a good one, and here's another one:
There are people who are really specialized in studying the dynamics of supernovae. But at the same time, dark energy was discovered by using supernovae as tools to measure distance, which revealed the accelerating expansion of the universe. So if we would like to use supernovae as tools, we need to actually understand them as precisely as possible. It turns out that the supernovae specialists, who were not interested in dark energy per se but interested in supernovae themselves, wanted to develop a new way of characterizing the behavior of supernovae. As a result of that work, the people who are interested in dark energy can use those studies of supernovae to understand systematic bias in their data.
"[The] IPMU was actually designed to be international, and it is supposed to offer a multinational and interdisciplinary approach to questions like dark matter and dark energy." – Hiroaki Aihara
The last example I can give you is string theory and mathematics. We have a lot of talks on research by our members, and during one a mathematician here named Todar Milanov discussed his desire to understand certain properties of geometry – this six-dimensional geometry that string theorists study. In his last slide, his presentation was really impressive. He listed a bunch of technical terms on that slide, and it turned out that half of them were either terminologies in physics or names of physicists. And this was a talk on a purely mathematical subject. So you can tell Todar kept getting a lot of inspiration from what people were doing in the physics world, and he was actually using some tools that were developed by physicists for his problem in geometry.
TKF: A single Grand Unified Theory that explains the physics of the universe on the smallest and largest scales eludes us today. String theory proposes a new explanation for matter and interactions at Planck scales – the very smallest. But it’s not yet testable. What are your goals for studying string theory, where you are now hiring additional faculty? And to what extent do your string theorists and experimentalists interact?

MURAYAMA: I view work on string theory as a great example of how mathematicians can be connected to data and experiments. The view is very simple: mathematicians develop the language for describing the physical laws of the universe, and string theorists use the language of mathematics to describe those laws. Once you have a description of physical laws, you can use them to describe real data, and that's what people like myself, called phenomenologists, do. Phenomenologists can talk to experimentalists to try to motivate new types of measurements and observations, and then work with them to interpret data. Experimentalists and observers, of course, talk directly to Mother Nature and give feedback to this entire chain of theorists. So that's the continuous spectrum I envision.
TKF: We often hear today that cosmology is at a turning point. For the first time in history, much of cosmology is a data-driven science – not just the realm of theorists in their offices or at a classroom chalkboard. We are now developing the technology to peer farther back in time, toward the very beginning of the universe. And we’re developing instruments to unveil the mysteries of dark matter and dark energy. How is this transition impacting your personal work at IPMU?
AIHARA: For me it's obvious. The HyperSuprimeCam that we were talking about is going to produce a large galaxy data sample. This is the first big survey using a large telescope like Subaru, so we can reach to the very deep universe. It’s going to produce an enormous amount of data for cosmology.
TKF: What are some of the other technologies involved that were just not available 10 or 20 years ago?
AIHARA: The first thing is the large aperture telescope, of course. Years ago, you didn't have any 8-meter aperture telescopes. You have to have a large aperture telescope to collect these photons from distant galaxies. And then, for this big survey, you have to have a wide field lens and a CCD for digital imaging. Also, big computers are necessary. Without these computers, you cannot process all this data. So it takes the combination of a large aperture telescope, a wide-field CCD camera and powerful computers. That has changed astronomy completely.
MURAYAMA: And computers are not just for processing data. They are for making theoretical predictions too.
TKF: Through these numerical simulations, such as the one IPMU did in 2011 to simulate the first stars?
MURAYAMA: Right. If you do observations, you would like to compare them to theoretical predictions so you can understand theories and test them. Without computers, you can't make predictions at the level of precision and accuracy that is needed to compare against data. The computers actually work both ways.

TKF: Dr. Suzuki, I would imagine the xenon detector that you're helping to develop for XMASS is a perfect example of how technology is allowing you to test your theories about dark matter.
SUZUKI: I think in the case of dark matter we know it's there but we don't know what it is. The detection efforts aim to find out what it is. I believe that it is very exciting for theorists. For our experiment we have adopted a new but very simple technology called a single phase detector, which we will easily scale up for the experiment in the future.
TKF: It's obvious that pursuing the biggest cosmological questions is a multi-national enterprise, not only financially but intellectually, with large teams of scientists hailing from institutions that each have their own strengths. What does IPMU uniquely offer, and where in the next decade do you think it will most make its mark?
AIHARA: IPMU was actually designed to be international, and it is supposed to offer a multinational and interdisciplinary approach to questions like dark matter and dark energy. This effort has to be international, and we designed the Institute to foster collaborations with Stanford University, Princeton University, U.C. Berkeley and other institutions to address big fundamental questions.
TKF: Looking forward a decade or two from now, how do you think IPMU will really make an impact?
MURAYAMA: I can only dream about these things, but I can imagine the XMASS program will find a signal of dark matter in its data. Immediately, the people at IPMU working on the theoretical side will want to come up with new theories to explain the data. These theories may involve extra dimensional space, so we will need to understand this tiny six dimensional geometry of space to actually work out predictions – and that's where we will have to rely on mathematicians. And these predictions will then get compared to the data again.
So this kind of closed circle, completed at a single research institute, is not something that you can imagine elsewhere in the world. Once we've seen incredibly exciting data, everybody from pure mathematicians to string theorists to particle theorists to astrophysicists will get together and think about how to understand that data, come up with a new theory, test it again with new data, and so on. Perhaps I am dreaming here, but that's the kind of dream we have.
TKF: Personally, what makes IPMU such a rewarding place to spend your career?
SUZUKI: IPMU is a completely new type of institute that will be a model for future scientific institutions in Japan. I hope that IPMU will produce important results that nobody today can imagine.
AIHARA: For me, this enterprise is exciting because we started from scratch. IPMU did not exist five years ago. Nobody was here, and now if you come to IPMU you enter this building and not even half of our scientists are Japanese. And they are all talking to each other. This never happened before. Something new is really starting, that's for sure.
MURAYAMA: Personally, I’ve also taken great pleasure in seeing the impact of the Institute on the people around this town and among people generally in Japan. I’ve also noticed how the high school kids are getting excited about science once again, and now I hear people actually talk about dark matter and dark energy – which I had never heard before. There is absolutely no doubt: IPMU is changing Japan.