Unraveling the Mystery of Right- and Left- “Handedness” in Biomolecules
by Adam Hadhazy
Kavli-Laukien Fellow Furkan Ozturk examines why life predominantly relies on amino acids, sugars, and other key ingredients with only certain mirror symmetry
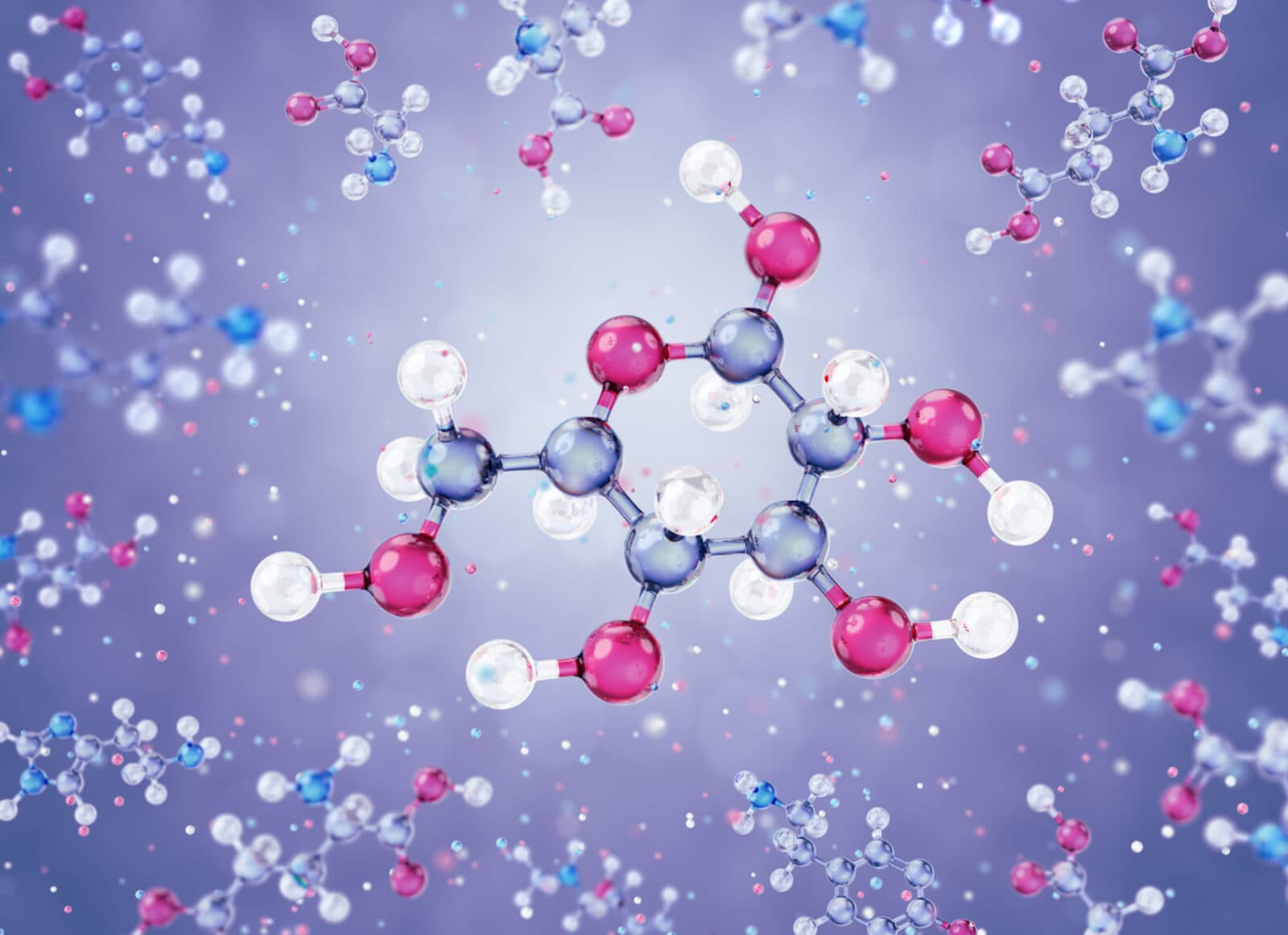
The Author
In September 2023, The Kavli Foundation and the Laukien Science Foundation announced a new fellowship program to support scholars in the Harvard Origins of Life Initiative. Through their research, Kavli-Laukien Postdoctoral Fellows are exploring how initial conditions on planets may enable life to emerge and subsequently evolve. 2024 Kavli-Laukien Fellow Furkan Ozturk is investigating life’s molecular “handedness.”
Life is a remarkably flexible phenomenon. From the driest deserts to the deepest oceanic abysses, creatures of the microscopic and even macroscopic variety occupy nearly every square inch of our planet.
Yet in one fundamental regard, life is profoundly inflexible. At the basic biochemical level, living systems predominantly use only one of two “handed” versions of molecules available in nature. Just like our left and right hands, many molecules can form structures that are mirror images of each other—the same in terms of composition, but oriented asymmetrically.
Take the amino acid blocking blocks of proteins, for example. In most cases, only the “left-handed” structures of amino acids are utilized by living cells. Similarly, the sugars in DNA are all distinctly “right-handed.” This choice of molecular mirror symmetry is not a trivial matter; if the oppositely handed versions are accidentally introduced into the body, for instance through faulty pharmaceuticals, toxicity or even death can follow.
The name for this intrinsic feature of life is homochirality—aptly derived from the Greek roots for “same hand.” Scientists have puzzled over homochirality since Louis Pasteur (of vaccine and food-preservation fame) first noted it back in 1848 when looking at mirror-imaged crystals of tartaric acid under a microscope.
The concept has likewise captivated Ozturk, who previously researched the physics of ultracold atoms for quantum simulation. Ozturk learned of the so-called homochirality problem in biology from a Science magazine article about the top 125 unresolved mysteries in science, which also ranked the origin of life itself among the biggest. Feeling that with his physics background he could approach these interrelated problems afresh, Ozturk is now continuing his work as a Kavli-Laukien Postdoctoral Fellow at the Origins of Life Initiative at Harvard University, where he initially came in 2018 to earn his Masters in atomic physics and where he completed his PhD earlier this year.
“Biology is very picky when it comes to chirality and we need to understand why that is and how it came to be,” says Ozturk. “It's a problem that I felt that I could attack with my skillset and background.”
Ozturk is building upon previous research he conducted that has hinted at the plausible origins of homochirality. The premise is that minerals with magnetic properties—such as the duly named magnetite—could have induced chirality on biomolecular building blocks growing on their magnetized surfaces. The setting for this interaction would have been bodies of water on early Earth, full of “primordial goo” of prebiotic molecules collected and mixed. The homochirality of life today would thus be a result of this physical chiral preference imparted to the chemistry of life from the get-go, roughly four billion years ago.
The underlying physical principle behind the homochirality mechanism is known as CISS (pronounced “sis”), which stands for chiral-induced spin selectivity. CISS, in turn, rests upon the physics of particles. All elementary particles have a property called spin. The term refers to a particle’s angular momentum, which physicists have analogized as the particle “spinning” in a clockwise or counterclockwise direction.
Electrons, for instance, can have one of two different spin states, referred to as “spin up” and “spin down.” When encountering chiral molecules, electrons with a particular spin will flow through that molecule more easily, whereas electrons with the opposite spin state will flow better through the oppositely handed version of the same molecule. In other words, spin up electrons move more efficiently through molecules with a certain handedness, and vice versa.
Ozturk further likens this description of CISS to the molecules having “selective channels,” explaining that “electrons only want to go through these channels if their spin state is right.” The outcome is that electrons of a certain spin state accumulate at the end of those chiral molecules with the corresponding channel.
Notably, the surfaces of magnetic materials also display these kinds of spin biases, because spin determines magnetic polarity (north or south). Just as with electric charge—another, more familiar elementary property of particles—opposites spin states attract and likes repel each other, the same as with magnets.
Accordingly, because chiral molecules are attracted to magnetic surfaces that possess opposite spin states, molecules of one chirality interact more effectively with magnetic surfaces of certain magnetization. The alike molecules thus pile up and lead to richer chemical interactions, forming crystal structures with identical mirror symmetry—including the building blocks of complex biomolecules, such as RNA—that are homochiral.
Ozturk and colleagues previously demonstrated this result in experiments involving ribo-aminooxazoline, a central RNA precursor. Moreover, in later work, the researchers suggested a pathway by which left-handed peptides (short chains of amino acids) could be formed from right-handed RNA analogs, addressing the related issue of opposite chirality observed in peptides and nucleic acids. While these findings represented significant breakthroughs in the quest to solve homochirality, they were reached in highly controlled, pristine laboratory conditions, using lab-made magnetic surfaces and chemically homogenous mixtures that are less representative of a natural primordial pond.
As Ozturk proceeds deeper into his Kavli-Laukien fellowship work, he will be exploring if this very same sort of homochirality induction could have occurred in conditions like those that theoretically existed on primordial Earth.
“In my PhD research, I verified that indeed magnetized surfaces can provide the necessary bias to select one chiral form over the other, using magnetite surfaces that I fabricated in the lab and relatively clean chemical mixtures,” says Ozturk. “Now the goal is to shift that work to more natural, geological environments that we expect on early Earth as opposed to pristine lab conditions.”
Those environments would have been lakes or streams with magnetic rocks on their beds and waters replete with key basic ingredients—including phosphate salts, ammonia, and cyanide perhaps—for cooking up simple biomolecules. Much of the energy for the ensuing chemistry would have been provided by ultraviolet light from the young Sun.
To simulate these conditions, Ozturk is developing a kind of prebiotic flow reactor that would bring all these materials and energy together, representing the complex and simultaneous interplay expected to have taken place. “We’re going from studying individual reactions one-by-one to studying mixtures of many compounds that combine and react on their own,” says Ozturk.
Looking ahead, Ozturk says that his research’s findings could not only help solve the interrelated mysteries of homochirality and the emergence of Earthly life, but also help guide astrobiologists and astronomers to the most suitable potential habitats for where alien life could have taken hold.
“If you better understand what kind of environmental conditions are needed, like whether you need magnetic mineral surfaces, or how many ponds or streams necessary to create these conditions, then you have a much better understanding of where to look for life elsewhere,” says Ozturk. “We are eager to run the experiments and let's see what happens.”