Wait-and-See and Go-and-Get: The Modes of Astrophysics
by Adam Hadhazy
Much information comes to us from the universe, but some we have to go gather ourselves
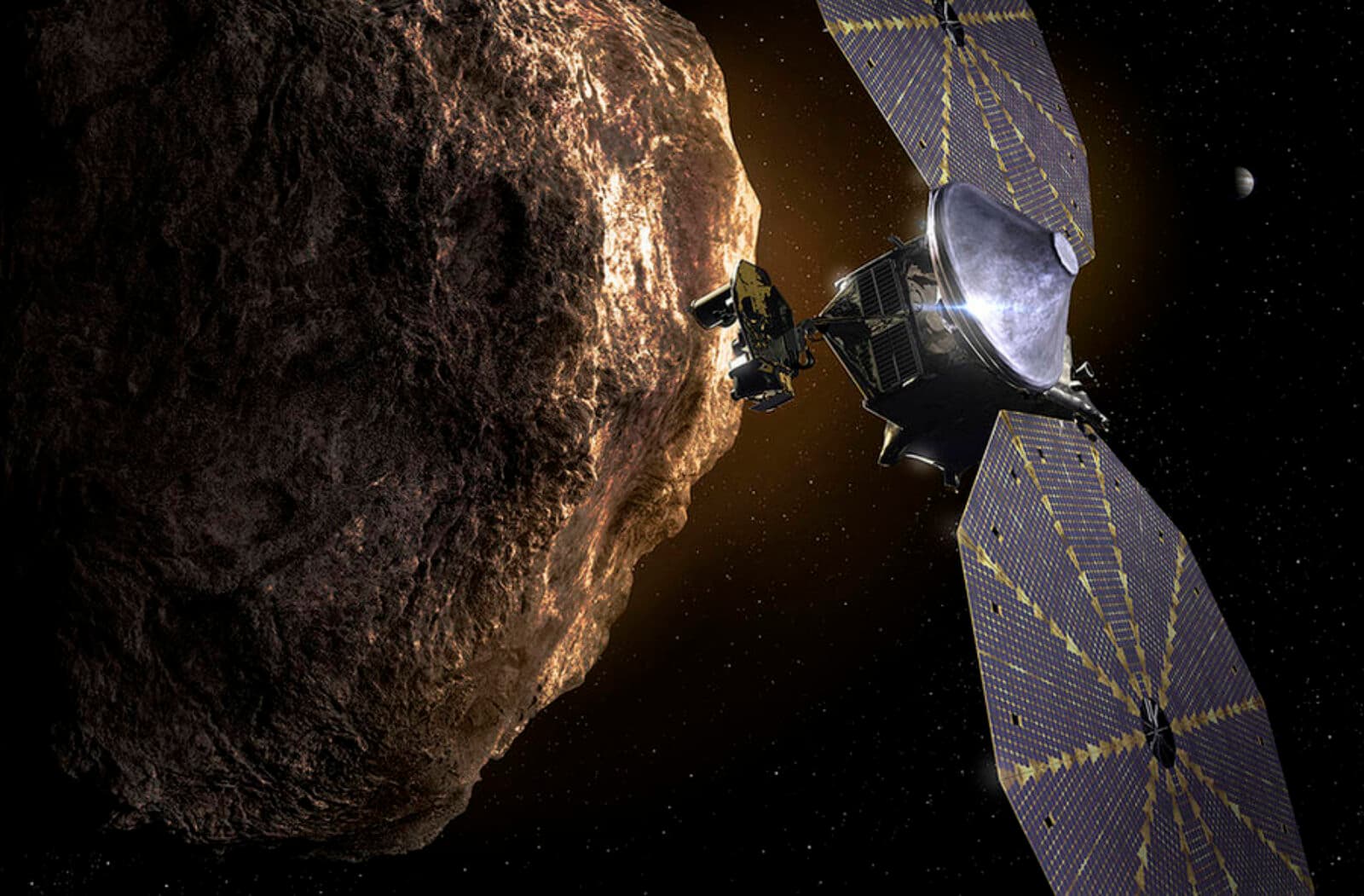
The Author
Unlike many other scientific disciplines, astrophysics can count on a certain generosity shown by nature. Our planet Earth is constantly graced by light arriving from celestial entities, from as close as the moon, the sun, the planets, and other objects in the solar system, outward to the stars throughout our galaxy, and farther and farther out to billions of galaxies, and even all the way back to the universe's oldest light, the afterglow of the Big Bang. Heavier bits of particles than light, known as cosmic rays, as well as the lightest particles of all, neutrinos, also make it all the way to us from across great cosmic divides. We've even figured out how to wrangle the ultra-subtle (by the time they reach us) ripples in the fabric of spacetime, dubbed gravitational waves, that are heaved out by cataclysmic events like black hole collisions. Quite simply, all we need do to catch these information-loaded incoming signals is to look and listen with our telescopes; build it, so to speak, and they will come. Earth's atmosphere does block out various forms of light and particles, so to catch everything the universe is throwing our way, we often send space telescopes aloft. Yet as well as this wait-and-see approach works, it is not enough for the business of planetary science, a field intimately tied into broader astrophysics. To really understand what's in our solar system—and extrapolate from there to all other space rocks and phenomena in all other solar systems—we have to go and get a closer look. Not enough light or other conveyer of information can reach us from the surfaces of solar system bodies to tell us what, say, the rocks on the moon or Mars are fully made of, or what Pluto actually looks like. We've accordingly sent astronauts to the moon and rovers to Mars, and sent a probe, called New Horizons, on a nine-year-voyage to finally see Pluto's face. This modus operandi continues now with the Lucy spacecraft, which will let us get up close and personal with the most numerous set of solar system objects yet to be visited, called the Trojan asteroids. Alas, the laws of physics practically limit this active form of exploration, of going-and-getting, to just our solar system; even a probe somehow launched with the energetically unobtainable velocities in remote spitting distance of the speed of light would take decades, if not centuries to reach the nearest stars and exoplanets. We must therefore continue to hone our abilities to reap the harvest of the bounteous cosmic energy and matter that freely come to us right here on Earth.
On the trail of inflation with the BICEP experiment
Inflation is a highly compelling theory that addresses multiple issues in cosmology, explaining how our universe looks the way it does. Researchers at the Kavli Institute for Particle Astrophysics and Cosmology (KIPAC) at Stanford University have been on the hunt for a predicted signal left by inflation on the oldest light in the universe, known as the cosmic microwave background, or CMB. Inflation supposes that the universe underwent a titanic expansion in size a mere trillionth of a trillionth of a trillionth into its existence during the Big Bang. This dramatic event should have generated ripples in spacetime, known as gravitational waves, that in turn would have left signatures in the CMB. A telescope located at the South Pole is running the so-called BICEP experiment, searching for these signatures. In the latest set of results from BICEP, the researchers announced they did not find the eagerly sought signatures. But, in the process, the researchers have constrained the properties these hypothetical waves would have. It's a null result, but such results are integral for knowing when something, has in fact, been discovered. The hunt will go on.

Demolition derby in a nascent solar system
The later stages of planetary formation are theorized to be violent periods, marked by cataclysmic collisions between worlds as solar systems settle down into a stable configuration. Our own moon is thought to be the product of such a collision between a nascent Earth and a Mars-sized body lost to history. Now researchers at the Massachusetts Institute of Technology's Kavli Institute for Astrophysics and Space Research (MKI) think they have spotted this same kind of planetary demolition derby happening in an alien solar system. That system, designated HD 172555, had been known to have large and varied dust signatures, originally attributed to a major planetary impact or an asteroid belt. The plot has recently thickened. In association with that dusty debris, MKI researchers and colleagues have newly reported the signature of a carbon monoxide gas ring. The presence of all that gas and dust suggest that two bodies collided, with one or both possessing considerable atmospheres. It's a remarkable new finding and once more shows that what happened here historically in our solar system is likely not unusual; whether that extends to the formation of life, though, remains a big question.
From neutrinos to gravitational waves
Takaaki Kajita has had a full scientific life. A Principal Investigator at the Kavli Institute for the Physics and Mathematics of the Universe since 2007, Kajita won a Nobel Prize Physics in 2015 for his breakthrough work showing that neutrinos spontaneously change a property called flavor, revealing that the squirrely subatomic particles do in fact have mass. Yet as a recent article in Physics World relates, despite his success with neutrinos, Kajita wanted to enter into a new field, and did so in 2008. He began working on the experiment that has become Japan's first gravitational-wave hunting instrument, known as KAGRA. Kajita, who now serves as the KAGRA project's principal investigator, is looking forward to the detector carrying on its observing campaign next year.

Neutron star mergers more of a goldmine than neutron star and black hole smashups
MKI researchers have provided new insights on the origins of natural chemical elements heavier than iron. The nuclear fusion in stars produces most of the elements lighter than iron, including familiar elements like carbon and oxygen. But nuclear fusion factories cannot get hot and compacted enough to go past iron. Researchers have thus worked out that the extreme conditions created when ultra-dense stellar remnants called neutron stars collide must be what leads to the formation or gold, platinum, and other heavy elements, generally up through uranium. Similarly extreme conditions also occur when neutron stars and even more compact objects, black holes, cataclysmically meet. An analysis of these two kinds of mergers, presented in a recent study, bears out that at least over the last 2.5 billion years of cosmic history, neutron star mergers have been the dominant way the universe has forged heavy elements. The novel findings will help in constraining how, where, and when heavy elements—which are rarer than lighter elements—appeared in and became distributed throughout the cosmos, and with certain abundances cropping up here on Earth.
Lucy mission delving into the Solar System's origins begins
In mid-October, NASA launched an exciting new mission, dubbed Lucy. The Lucy spacecraft will make humanity's first-ever visit to the Trojan asteroids—enigmatic space rocks clustered in two bunches in front of and behind the planet Jupiter in its orbit. The Trojans are pristine time capsules from the early solar system, preserving chemical evidence of the conditions when our local worlds took shape over four eons ago. The project scientist for the Lucy mission is Richard Binzel, who is an affiliated faculty member of MKI. He points out that materials visible on the asteroids Lucy will visit could date back 4.56 billion years, right to the very dawn of our solar system and older than any samples we could study from the moon or find on Earth. The Trojans could shed light on the origin of carbon-containing compounds, so-called organics, necessary for the rise of life. The spacecraft will reach its first of several Trojan targets in 2027.
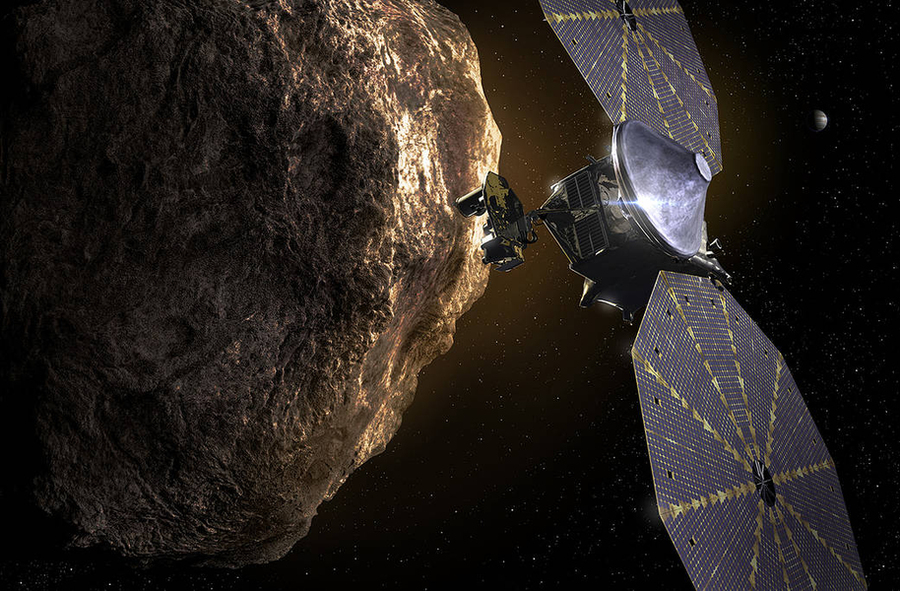