Water from Desert Air
by Alan S. Brown
Porous crystal make it possible to pull water from desert air, and other nanoscience advances
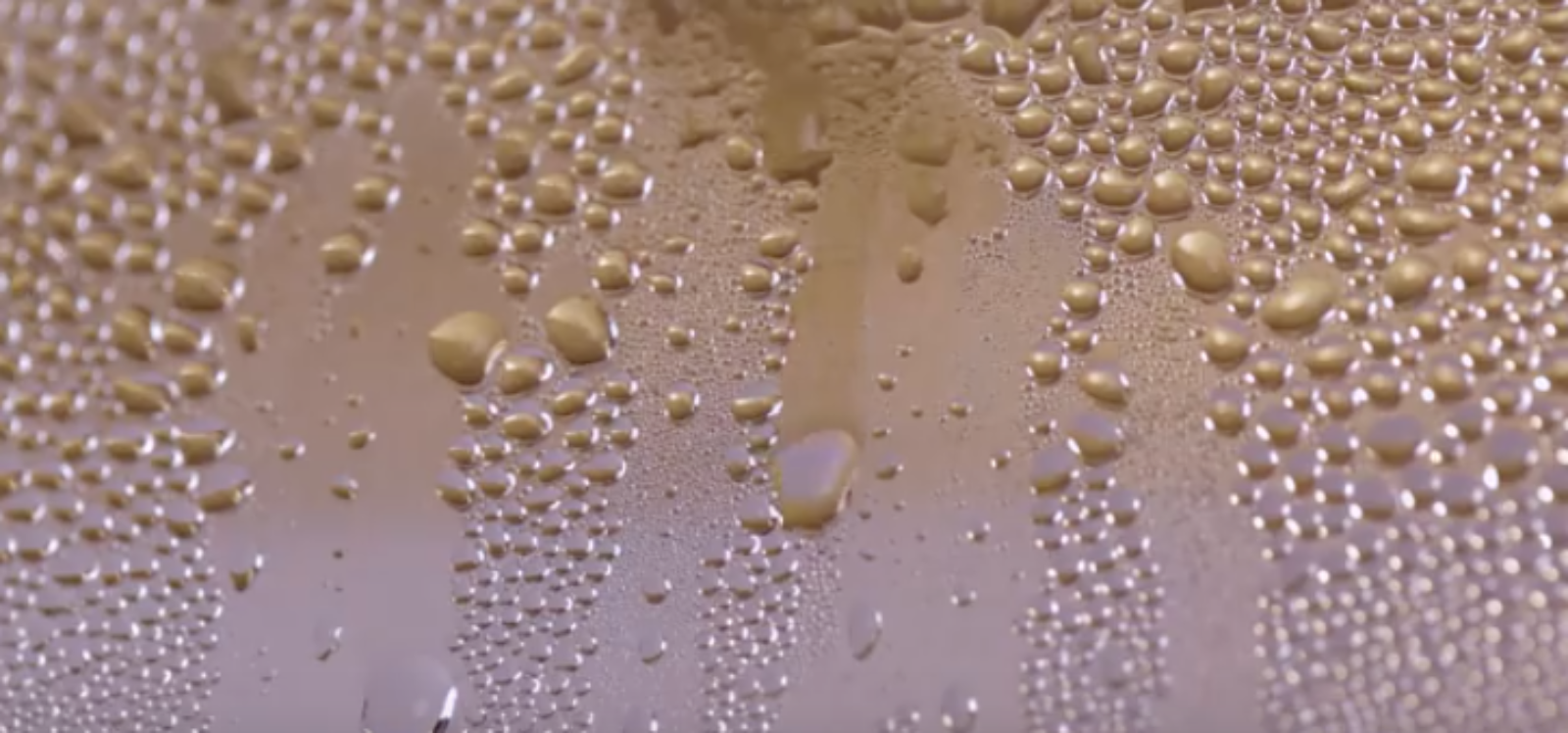
The Author
The Researcher
From the outside, scientific advances often look like the smallest of small victories. Who, after all, really cares if a robot—and we’re using the term loosely here—can roll itself into a wheel and move without any source of power? Or whether there is a better way to twist a sheet of graphene or examine the workings of your cells’ skeleton? Yet those small advances may one day bloom into something incredible. Take, for example, Omar Yaghi, co-director of the Kavli Energy NanoScience Institute at Berkeley. Twenty years ago, he began playing with an unusually porous crystal made (like a Tinker Toy creation) of metal hubs and organic rods. It sounded pretty obscure. This month, he announced how a small device based on those materials could pull enough water from the desert in a single day for a family to drink, cook, and bathe. Which makes our point—no one knows where a scientific advance will lead.
- Water from desert air.
Several years ago, Omar Yaghi, co-director of the Kavli Energy NanoScience Institute at Berkeley, showed the world a device without any moving parts that could pull water out of the desert air. Now he has something even better to show: a water harvester that is nearly ready for commercialization. At its core is an incredibly porous molecule (called a metal-organic framework, or MOF) that strips water from air as it passes through, then releases it when heated. It also includes a solar cell and battery to speed the process. In tests in California’s Mojave Desert, the harvester reliably produced 0.7 liters per kilogram of MOF per day, 10 times better than his previous device. Yaghi estimates a harvester the size of a small refrigerator would provide enough water for a family to drink, cook and shower.
- Magic angle puts new twist on superconductors
Superconductivity is an elusive dream with big payoffs, like electrical transmission without losses and quantum computers. Unfortunately, most superconductors are hard to work with. Now, there is a new contender—graphene. Last year, MIT researchers discovered that twisting a layer of graphene 1.1 percent can suddenly turn the material into a superconductor. Graphene is already used for quantum computer research, and adding superconductivity just makes the deal sweeter. Lots of researchers have jumped on this. Now, a team led by Stevan Nadj-Perge of Caltech’s Kavli Nanoscience Institute, has developed a way to make twists more easily—and image the graphene’s electronic properties at atomic-length scales. This promises to help scientists try to understand why twisting works so we can better control it.
- Your cells’ skeleton closets.
What keeps your cells from oozing all over the place? It turns out they have skeletons to hold them together. They also alter their shape to move, ingest nutrients, and divide. These cytoskeletons are made up of nanosized microtubules that are 1,000 times thinner than a human hair (about 60-80 nanometers) and 10,000 nanometers long. Their size makes it incredibly difficult for researchers to study their inner workings. Now a team that includes Rob Phillips, a member of Caltech’s Kavli Nanoscience Institute, has developed a way to grow microtubules in test tubes—and shape them into interesting structures. Not will this help us investigate how they work, and may one day lead to bioinspired therapy microrobots and mix and manipulate liquids in biomedical tests.

- Kirigami slices its way to any shape.
Researchers have a growing fascination with kirigami, an Oriental artform that resembles origami but uses cuts instead of folds. Now researchers from the lab of L. Mahadevan, a member of Harvard’s Kavli Institute of Bionano Science and Technology, has developed a way to determine the slices needed to turn a sheet of paper into any two- or three-dimensional object. The team uncovered the basic mathematical principles underlying kirigami, then used them to create algorithms that work backwards from a shape to the location, length, number, and orientation of cuts needed to make it. One possible use: Turn flat pieces of silicon into electronically controlled moving machines.

- Rollbot rolls along without power.
To move, robots need power. It might come from an electrical socket, a battery, or a solar cell, but it needs to come from somewhere, right? Maybe not. Researchers at Kavli Institutes at Harvard (Jennifer Lewis) and Caltech (Chiara Daraio) have collaborated on a shape-shifting robot goes through some interesting transformations without any external power source. Place the flat robot on a plate heated to about 200 degrees C, and the heat will cause it turn into a pentagonal wheel. It has a hinged flap on each face. When heated by the plate, the flap pushes down, pushing the wheel forward. When the next face reaches the plate, its flap does the same. The result: a moving robot powered by a material’s reaction to changes in temperature. The rollbot is not yet doing useful work, but this new way of thinking of locomotion could open the door to new ideas for propulsion in the future.