Where the Quantum World Begins
by Alan S. Brown
Research highlights from Kavli Nanoscience Institutes
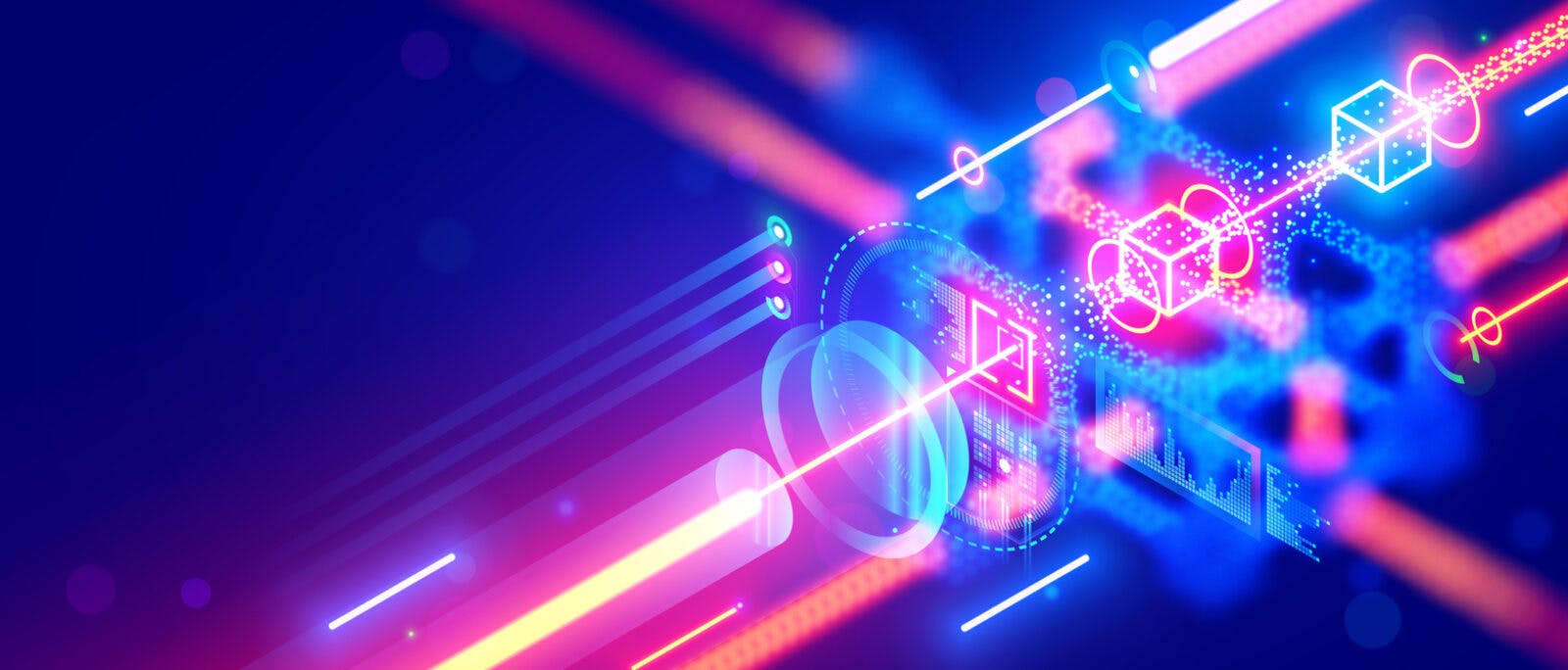
The Author
The latest research highlights from Kavli Nanoscience Institutes feature a few significant firsts. At the Kavli Energy NanoScience Institute at UC Berkeley, researchers have found a way to mechanically interlink millions of molecules, opening the door to materials that flex and stiffen in response to different stimuli. At the Kavli Institute at Cornell for Nanoscale Science, researchers have discovered a way to vary a crystal’s symmetry—and its electronic, optical, and other properties—using tiny amounts of voltage. We also feature a discussion about a table-top experiment to measure quantum gravity, how artificial intelligence can be applied to help in the production of nanomedicines, and new insights into the complexity of bacterial membranes actually work.
Probing where the quantum world begins
We all know that the laws that govern the quantum world differ from those that enable us to predict what occurs in our everyday life. But we know surprisingly little about how these two worlds interact at their interface. One way to discover more is to attempt to unify quantum physics with the macroscopic world of gravity and space. That is the topic of discussion between Rana Adhikari, a member of the Kavli Nanoscience Institute at Caltech, and Kathryn Zurek, a theoretical physicist at the same institute. Along with collaborators, they are building a table-top experiment to detect the signatures of quantum gravity. Why gravity? Today, quantum theory does a good job explaining such fundamental forces as electromagnetism (which we see in the macro world) and the strong and weak forces that govern the behavior of subatomic particles at the quantum level in ways that have macro repercussions. Gravity, however, remains an outlier. We can see and measure it in the macro world, but no one has found evidence of it at the quantum level. Adhikari and Zurek hope to see artifacts of quantum gravity by looking for small-scale ripples in spacetime. The experiment itself works a bit like LIGO, the laser interferometer gravitational-wave observatories operated by Caltech and MIT, but at a much smaller scale. To learn more about this remarkable quest, listen to Caltech’s 45-minute interview with Adhikari and Zurek.
One step to molecular “chain mail” sheets
Seven years ago, French chemist Jean-Pierre Sauvage shared a Nobel Prize for synthesizing the first catenane, which consisted of two chemical rings interlocked with one another mechanically, not unlike medieval chain mail. Since they were not locked in place by chemical bonds, they could move freely yet still retain the strength and stiffness of the original molecules. Researchers speculated that sheets of catenanes would make excellent reinforcements for composites without the brittleness of conventional fracture-prone reinforcing fibers. Unfortunately, catenanes are hard to synthesize: adding each ring took an entirely new chemical reaction. Now, a research team led by Omar Yaghi, codirector of the Kavli Energy NanoScience Institute at UC Berkeley, has found a way to synthesize millions of interlocked catenanes with a single chemical step. The work, which builds on Yaghi’s covalent organic frameworks (COFs), synthesizes two boomerang-shaped molecules covalently bonded to a copper atom where they cross. The synthesis process yields a porous 3-D network of interlocking polyhedral molecules whose six arms interlock to form an extended framework. By chemically modifying the molecules, Yaghi believes he can not only make good reinforcements, but create systems that flex or stiffen in response to different stimuli.

Switch crystal symmetry with the flip of a switch
For the first time, researchers have shown that they can vary a crystal’s symmetry—and its electronic, optical and other properties—using voltage. Until now, voltage has broken or removed symmetry, rather than switched it. The breakthrough could lead to entirely new classes of low-power, nonvolatile semiconductors for computer memory and logic devices. Two Kavli Institute at Cornell for Nanoscale Science (KIC) members were senior leaders on the project: Darrell Schlom, who produced the materials in his lab, and KIC co-director David Muller, who used his ground-breaking electron microscope pixel array detector (EMPAD) system to image atoms and elucidate the polar and nonpolar phases of material. The material itself consists of a few atomic layers of bismuth ferrite, a ferroelectric with the highest polarization of any known material, and terbium scandate, which is not a ferroelectric. Because each layer of the superlattice is so thin, it has a major influence on the other layer. By adjusting layer thickness, the researchers perfectly balance these influences. This enables a small electric current to trigger a large change in symmetry. This boosts bismuth ferrite’s resistivity by five orders of magnitude, turning it into a semiconductor from an insulator, and changes the superlattice’s nonlinear optical response by more than three orders of magnitude. Next, researchers plan to investigate the material’s magnetic response.
AI drives nanomedicine engineering
What happens when you apply artificial intelligence to nanomedicine engineering? The National Science Foundation has awarded $3 million to Kavli Institute at Cornell member Nicholas Abbot, an expert in liquid crystals, and Cornell Rong Yang, an AI researcher, to find out. Today, nanoparticles are an emerging but problematic element in the development of targeted drugs. Pharmaceutical makers attach medicines to chemically modified nanoparticles that bind to specific tissues in the body. It may take years or even decades to develop ways to manufacture these particles consistently and at scale; but Yang believes AI could help simplify this process, but better data on these tiny molecules is needed. Enter Abbot, who will use the optical properties of liquid crystal polymers (LCPs) to provide the data Yang needs. The approach is to dissolve the nanoparticles in LCPs, which are used in optical displays. As the two materials interact, the optical response of the LCPs will provide data about nanoparticlemolecular weight, size, and morphology. That information will be used by AI algorithms to better model how to synthesize the nanoparticles during assembly.
Rethinking bacterial membranes
Until recently, biologists assumed the outer membrane of Gram-negative bacteria consisted of a gel-like layer of lipids containing randomly distributed proteins. Now that researchers can image live cells, such as E. coli, they are finding that this is not the case. Instead, the proteins on bacterial surfaces sit in the center of hexagonal-like lipid structures. This is interesting because bacterial membranes do more than just hold the cell together and let in nutrients and expel waste. They also are the organism’s first line of defense, ejecting antibiotics, hydrolyzing antimicrobial peptides, and controlling adherence to surfaces to form colonies. Understanding membranes could help researchers develop more effective antibiotics. In a recent video, Abraham Oluwole of the Kavli Institute for Nanoscience Discovery at the University of Oxford interviewed his collaborator, Melissa Webby, about her four-year program to probe the nature of these outer layer membranes. Since proteins and lipids respond very differently to advanced measurement techniques, it took a lot of collaboration among Kavli members and other Oxford researchers to unravel the mysteries of these biochemical systems.