X-ray Light of a Certain Bent
Beyond just scooping up ever more loads of light, researchers are after certain observable properties to advance astrophysics
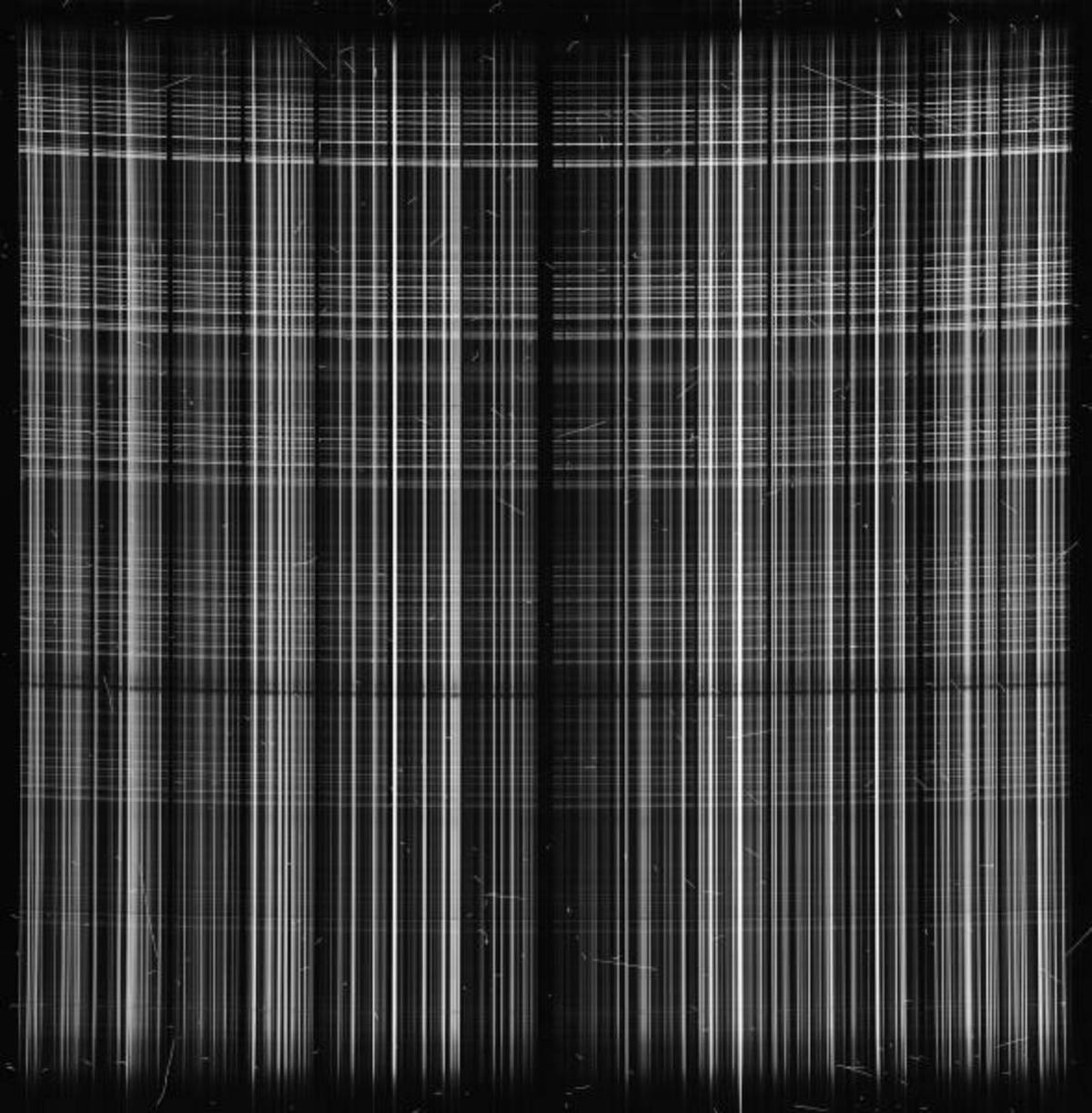
When it comes to astronomical and astrophysical observations, more is of course the merrier; more light and more particles mean more data and ultimately more science. To this end, gathering up as much and as many kinds of emissions as possible from cosmic sources via bigger telescopes, higher-sensitivity detectors, and so on, has accordingly long been the name of the game. Within this overarching goal of "more," though, are targeted goals of "more of a certain thing," or even brand-new things. A compelling recent example of squeezing more understanding out of what could simply have been "more" is NASA's Imaging X-Ray Polarimetry Explorer (IXPE). This satellite launched about a year ago in early December 2021 as the first spacecraft dedicated to measuring the polarization of cosmic X-ray light. Quite a number of observatories that collect X-rays have been launched over the past 60 years, and they've all had to be put into space because Earth's atmosphere blocks this high energy form of light. Now IXPE is looking at a particular characteristic of X-ray light, polarization, that hasn't gotten its due. Polarization is somewhat familiar to us in the context of polarized sunglasses. Technically, polarization refers to a property of transverse waves, like light, which vibrate perpendicularly to their direction of travel; an analogy is a plucked guitar string, where a wave propagates down the string in a certain direction while the string vibrates sideways. Detecting the polarization of X-rays is key for mapping the magnetic fields of astrophysical objects including the remnants of massive stars, such as neutron stars and their pulsar and magnetar cousins, as well as black holes. Researchers will also be able to far better study clouds of matter that are bright in X-rays toward the center of our galaxy. Initial findings with IXPE have started rolling out, and Kavli astrophysics institute members are just some of the scientists internationally that have taken advantage of the important new "more" that this instrument is making available.
Digging deep on how spinning, departed stars crank out X-rays
Pulsars—the spinning, radiation-spewing remnants of massive stars—have long fascinated astrophysicists. And despite tremendous progress in understanding these objects, many questions remain. One of those questions is how exactly high-energy light from pulsars is generated. NASA's Imaging X-Ray Polarimetry Explorer (IXPE) is now helping to answer this question—admittedly by posing fresh questions. Researchers, including members of the Massachusetts Institute of Technology's Kavli Institute for Astrophysics and Space Research (MKI), are surprised that IXPE has shown that X-rays from pulsars can be very weakly polarized compared to theoretical expectations. The results could be limited to the pulsars studied and not indicative of these objects overall. Nevertheless, scientists expect to have to revisit and revise their ideas on how these extreme objects can spit out high-energy light.
The nitty-gritty of black hole jets
IXPE is moving astrophysics forward on other fronts, too. Researchers at the Kavli Institute for Particle Astrophysics and Cosmology (KIPAC) at Stanford University are involved in a study that is helping answer a 40-year-old mystery about how particles are accelerated to extremely high energies in jets shot out by supermassive black holes. Observations of x-rays, visible light, and radio waves emitted from these jets that reach all the way to Earth showed that all these wavelengths of light were polarized in the same direction as the jet. Matching those observations to models show that particle acceleration is likely caused by a shock wave within the jet. Further verifying this arrangement could enable scientists to more thoroughly understand the nitty-gritty of high-energy astrophysics in environments unlike anything directly observable in Earthly laboratories.
New milestone for the starlight-harvesting Prime Focus Spectrograph
An important step forward has been taken for the Prime Focus Spectrograph (PFS), an instrument placed on the Subaru Telescope in Hawaii. The international collaboration behind PFS—which includes members of the Kavli Institute for the Physics and Mathematics of the Universe (Kavli IPMU) at Tokyo University—announced recently that they had successfully used the instrument to gather spectra of stars. These spectra are detailed observations of the star's light that reveal information such as the star's makeup and distance. PFS will ultimately be used to take collective starlight spectra from millions of galaxies in order to probe astrophysical mysteries such as dark matter and dark energy.
Expanding the hunt for ghostly neutrinos in Greenland
Nearly massless, stunningly copious, and with properties in defiance of the highly successful standard model of particle physics, neutrinos are also unparalleled astrophysical messengers. Because the oddball particles travel cleanly through space from their cosmic sources, the particles can reveal much about their distant sources' fundamental astrophysical processes.
Researchers at the Kavli Institute for Cosmological Physics (KICP) at the University of Chicago are involved in an effort that's building a new neutrino-collecting array in Greenland, called Radio Neutrino Observatory (RNO-G). The detector will be among the world's largest and most sensitive for high-energy neutrinos and should help advance the state of knowledge of numerous astrophysical fields.
Why so handed? Researchers connect space radiation to life's intrinsic skew.
A thread running through all cosmological and astrophysical inquiry, no matter how abstract such inquiry may seem, is us—namely, how everything that ever has been has somehow culminated in the formation of life, and specifically human life. To this end, KIPAC researchers are explicitly working on solving a biochemical mystery of life that likely has a fascinating astrophysical connection. Key molecules for life—for example, amino acids—puzzlingly display a "handedness," or specific chirality, in their structure. The homochirality of such biomolecules means that while the molecules can be left- or right-handed, those used by life are only of one variety. A theory is that the soup of prebiotic molecules that eventually formed biomolecules on Earth were exposed to cosmic radiation (ultraviolet starlight, in this case) that preferentially broke down one handedness versus the other. However, it has been difficult to sort out how enough of this asymmetry could have been plausibly produced. KIPAC researchers and colleagues are thus taking another tack by examining whether cosmic radiation could have instead acted as a pressure on larger chains of biomolecules. The radiation at play in this case would be muons—heavier cousins of electrons formed when cosmic rays strike our atmosphere. The ongoing work could at last explain a perplexing feature of our most fundamental biological selves.