The Language of the Cell
by Alan S. Brown
mRNA vaccines are only the beginning for bionanoscience
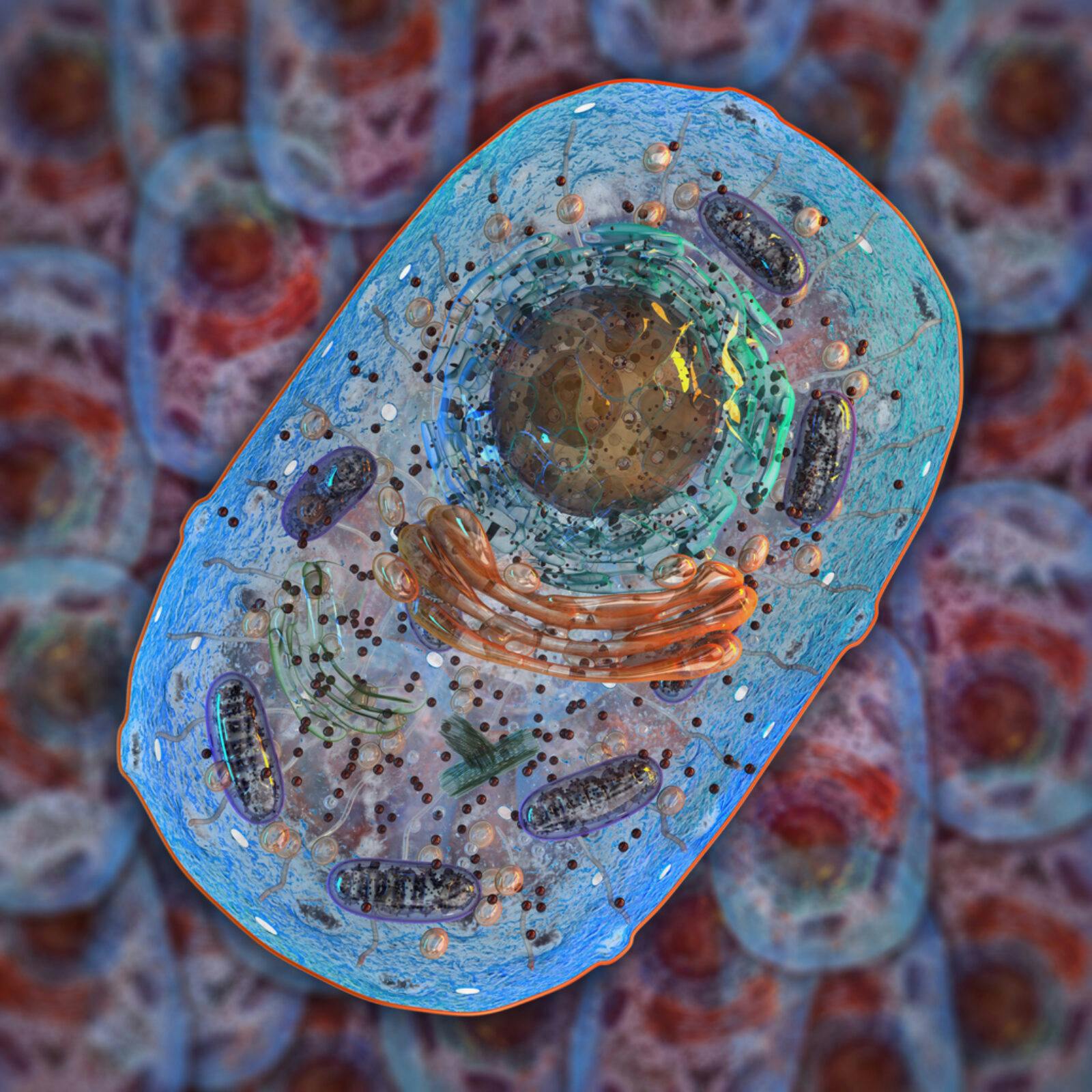
The Author
The Researcher
The new Covid-19 vaccines are truly revolutionary. Based on messenger RNA (mRNA), they evoke an immune response as strong as the best conventional vaccines—upwards of 90 percent—and are faster and less costly to manufacture. Yet mRNA has the potential to do far more than protect against Covid-19. It could help fight influenza, HIV, and other infections as well as cancer and genetic disorders, such as sickle cell anemia and cystic fibrosis.
Vaccines based on mRNA instruct cells to make proteins that make up the spikes of the coronavirus. Cells identify those proteins as foreign bodies and build antibodies to defend against them. When the body sees those proteins on a corona virus spike, those antibodies immediately spring into action and attack.
Those vaccines were not invented in a vacuum. It took an army of academic researchers decades to decode mRNA’s role in the cell and learn how to harness it for medicines.
The investigators who developed the vaccine benefited from years of fundamental research by interdisciplinary teams of scientists, including nanoscientists.
Chirlmin Joo, co-director of the Kavli Institute of Nanoscience at Delft University of Technology in the Netherlands
“Those scientists were the ones who unraveled how cells generate mRNA, recognize it, and translate it into proteins, and how we can regulate these processes. The nanoscientists discovered ways to modify or encapsulate RNA so they can deliver it into a cell without the body immediately eliminating it.”
The members of the Kavli Institute’s Department of Bionanoscience contributed to some of that fundamental mRNA research. Yet their research is by no means limited to mRNA.
“Our goal,” Joo said, “is to interpret the language of the cell. We want to understand the genetic code at its most fundamental level and then learn to use those mechanisms to make the cell do new and useful things.”
By learning to modify cells, Kavli researchers could lay the groundwork for new advances medicine, biology, agriculture, and chemistry every bit as revolutionary as mRNA. But first, they must learn how to entice nature’s miniscule building blocks to give up their biggest secrets.
Collaborators
The Kavli Institute of Nanoscience at Delft is divided into three groups, the Department of Bionanoscience, the Department of Quantum Nanoscience, and QuTech, which focuses on quantum computers.
“Diversity is our strong point,” Joo said. “Since we started the Department of Bionanoscience in 2010, we have had active discussions about whether to organize around certain topics or go for excellence in research regardless of a researcher’s focus. We have decided we want the best of the best researchers because, at the nanoscale, there are so many different systems. The knowledge needed to investigate them has to be very broad. You cannot spend your career looking at one cellular system or using one particular research technique. You have to collaborate, be open minded, constantly on the lookout for new technologies.”
For the Bionanoscience Department, that meant recruiting scholars with backgrounds in cell biology, molecular biology, and structural biology as well as chemistry and physics. The latter are especially well-versed in teasing out answers from incredibly hard-to-measure molecules.
A typical protein, for example, is only about three to six nanometers in diameter, so small that it takes 25,000 of them to bridge the width of a human hair. DNA is two meters long, but only two nanometers wide and folds into a bundle that fits inside the cell nucleus. In fact, many biomolecules fold themselves in complex ways and change shape when they are active.
To unveil the secrets of such small and complex molecules, Kavli researchers often turn to techniques originally developed by physicists to probe nanomaterials. One example is single-molecule spectroscopy, which provides information on the structure and function of individual molecules, like proteins and RNA. The technique originally worked only under extreme conditions—just a few degrees above absolute zero and no moisture. Over time, physicists and biologists tamed it so they could use it to investigate biomolecules at room temperature in water.
In addition to collaborating their bionanoscience colleagues, Kavli investigators sometimes also work with instrument experts from the quantum groups.
Joo, for example, turned to a quantum nanoscience expert in graphene to develop a way to study individual protein molecules. Graphene, a sheet of carbon only one atom thick, has unusual mechanical properties. It is so thin, it vibrates all the time. By measuring the changes in those vibrations when he places a single protein — which weighs less than an attogram (10-18 g) — on it, Joo believes he can detect a difference in mass down to a single proton.
Thanks to this type of fusion between diverse experts using specialized instruments, the members of the Bionanoscience Department have grown increasingly ambitious in the type of problems they have chosen to attack.
Synthetic Biology
Historically, the Bionanoscience Department started with single-molecule biophysics, Joo said. Advances in instruments enabled investigators unravel how, for example, the cell compacts a two-meter-long strand of DNA into the cell’s nucleus but makes it readily accessible when the cell needs to make proteins.
With their growing insight into individual molecules, Kavli researchers set their sights on the myriad processes taking place within the cell. This called for different types of imaging methods, such as enhanced electron microscopes with software that improves their resolution still further.
The best way to truly understand a cell, however, is to try to create one from scratch. Kavli researchers have done exactly that, forming a cytoskeleton-like structure within a droplet of water based on a well-chosen set of purified proteins. This synthetic network resembles those found in natural cells, though it does not yet mimic the multi-tasking capability of natural cytoskeletons.
These artificial structures serve as a type of bionano lab bench. As investigators grow new and better artificial cytoskeletons, they learn more about how which proteins are needed and in what order to complete the task.
Now, Kavli Delft researchers are going one step further and attempting to build a minimal cell—one able to perform all basic functions of life. Bionanoscience Department chair Marileen Dogterom is leading a national multi-university consortium, Building a Synthetic Cell (BaSyC), that hopes to fabricate an artificial cell from the ground up within the next 10 years. The timeframe may be optimistic, but the simple fact that scientists already have enough knowledge to begin undertaking this task is breathtaking.
Perspective
Even getting close would open the door to new insights and new ways to study cellular mechanisms. “If you want to see how genetic networks work,” Joo said, “you don’t need a cell that does everything. You can just reconstitute the few components that you are interested in. Or if you want to study some aspect of its metabolism or screen medicines, you could mimic those essential components and see how they react.”
What does the future hold for bionanoscience research? “Nobody has an answer for that,” Joo said. On one hand, he is certain that investigators will continue to develop existing new technologies, like single-molecule sequencing and new methods for genetic engineering.
On the other hand, he said, even newer trends and technologies are likely to reshape how research is done in the future. One of them involves how research takes place.
“The interface between academia and industry is becoming blurry,” he said. “The mRNA vaccine is a perfect example. Academia was pushing RNA medicines so hard, but in the end, it took industry to make it happen.” He predicts researchers working on fundamental problems in bionanoscience are likely to find themselves more involved with private companies in the future.
He also believes artificial intelligence will play an important role in the future. One example he cites is Google’s AlphaFold, which can predict a protein’s three-dimensional shape based on its amino acid sequence. This could open the door to a deeper understanding of proteins and the development of more powerful medicines.
Fifteen years ago, Joo laughed when he heard computer scientists talk about building virtual cells. Now he believes this could happen sooner rather than later.
“What if we could model how proteins interact with other proteins or with DNA and RNA,” Joo said. “We could mimic a cell computationally and predict its behavior and what types of drugs we need to fight a specific disease.”
The new mRNA vaccines have underscored the importance of bionanoscience this year. Yet the field has only begun to achieve its full potential.